For decades anatomy textbooks taught that the two most complicated systems in the body—the brain and the immune system— existed in almost complete isolation from each other. By all accounts, the brain focused on the business of operating the body, and the immune system focused on defending it. In healthy individuals, the twain never met. Only in certain cases of disease or trauma did cells from the immune system enter the brain, and when they did so, it was to attack.
But in recent years a rush of new findings has revolutionized scientists’ understanding of the two systems. Mounting evidence indicates that the brain and the immune system interact routinely, both in sickness and in health. The immune system can help support an injured brain, for example. It also plays a role in helping the brain to cope with stress and aids such essential brain functions as learning and social behavior. What is more, the immune system might qualify as a kind of surveillance organ that detects microorganisms in and around the body and informs the brain about them, much as our eyes relay visual information and our ears transmit auditory signals. In other words, the brain and immune system do not just cross paths more often than previously thought—they are thoroughly entwined.
Researchers are still in the early stages of studying this burgeoning new field of neuroimmunology. But already it is becoming clear that the brain’s response to immunological information and how that information controls and affects brain circuitry could be the key to understanding many neurological disorders—from autism to Alzheimer’s—and developing new therapies for them. Efforts to treat such conditions have typically met with disappointing results because most drugs cannot easily penetrate the brain. The findings from neuroimmunology raise the tantalizing possibility that targeting the immune system might be a more effective tactic.
Received Wisdom
To understand the significance of these discoveries, it helps to know a bit about how the brain and immune system are structured and how they work. The brain is our supercomputer and master regulator. Working with the spinal cord and several cranial nerves, which together constitute the central nervous system (CNS), it controls all the body’s functions. Given the vast scope of the brain’s responsibility, it is perhaps no surprise that the organ is incredibly intricate. Its basic functional units are neurons, which occupy roughly half of the brain. The human brain contains an estimated 100 billion neurons interlinked by approximately 100 trillion connections called synapses. The neurons, along with various types of nonneuronal cells called glia, make up the brain’s parenchyma, the functional tissue responsible for processing information. Other key players include stromal cells, which physically support the parenchymal tissues, and endothelial cells, which compose the blood vessels that supply the brain and form the blood-brain barrier, which limits the passage of substances from other parts of the body into the brain.
For its part, the immune system has two major components, innate immunity and adaptive immunity. Innate immunity is the more primitive element, having evolved about a billion years ago in the first cells to detect and dispatch enemy forces quickly but without much precision. It is the body’s first line of defense against pathogens, consisting of physical and chemical barriers to them, as well as cells that kill them. Innate immunity initiates the inflammatory response, in which white blood cells swarm the site of infection and churn out proteins that induce heat and swelling to confine and destroy pathogens. Adaptive immunity, which evolved after the innate component, consists mainly of cells called T lymphocytes and B lymphocytes, which can recognize a specific pathogen and mount a correspondingly targeted attack against it. In a perfect world, all adaptive immune cells would take aim only at external pathogens and would not touch the body’s own proteins or cells. But in about 1 percent of the population, adaptive immunity loses control and attacks cells in the individual’s own tissues, causing autoimmune diseases such as multiple sclerosis, arthritis and certain forms of diabetes, among many others. Still, the system has an impressive success rate, targeting foreign invaders exclusively in some 99 percent of individuals.
.png)
Researchers long thought that the immune system worked by simply distinguishing an organism’s own constituents from nonself ones. But eventually more complex theories began to emerge. In the 1990s Polly Matzinger of the National Institute of Allergy and Infectious Diseases proposed that the immune system recognizes not only foreign invaders but also damage to tissues. This notion gained support after the subsequent identification of molecules that are released by injured, infected or otherwise damaged tissues. These molecules attract the attention of the immune cells, triggering a cascade of events that lead to activation of the immune system, recruitment of immune cells to the site of injury, and elimination (or at least an attempt at elimination) of the alarm-causing invader or injury. In addition, experiments have found that suppression of adaptive immunity accelerates the development and growth of tumors and slows down the healing process in damaged tissues. Such findings show that the immune system—once considered to be laser-focused on protecting the body from foreign invaders—actually has a far greater purview: regulating the body’s tissues to help them maintain equilibrium in the face of all manner of insults, whether from without or within.
But until recently, scientists were quite sure that this purview did not extend to the brain. As early as the 1920s, researchers observed that although the healthy brain harbors immune cells native to the CNS called microglia, immune cells from elsewhere in the body (so-called peripheral immune cells) are not usually found there. The blood-brain barrier keeps them out. In the 1940s biologist Peter Medawar, who won a Nobel Prize for his research, showed that the body is slower to reject foreign tissue grafted onto the brain than grafts placed elsewhere in the body. The brain was “immune privileged,” Medawar argued, impervious to the immune system. Peripheral immune cells do appear in the parenchyma and spinal cord of patients with brain infections or injuries, however. And mouse studies demonstrate that these cells cause the debilitating paralysis associated with the disease. Based on such findings, scientists suggested that the brain and immune system have nothing to do with one another except in cases of pathologies that allow immune cells to enter the CNS and wage war on neurons.
(Exactly how the immune cells breach the blood-brain barrier in such instances is uncertain. But it may be that the barrier gets activated during brain diseases in ways that allow immune cells to cross over. In a seminal study published in 1992, Lawrence Steinman of Stanford University and his colleagues found that in mice with a condition similar to multiple sclerosis, peripheral immune cells make a protein called α4β1 integrin that allows them to penetrate the barrier. A drug that inhibits the interaction between the integrin and the endothelial cells, Tysabri, is one of the most potent treatments for multiple sclerosis patients.)
The theory that the brain and immune system lead separate lives prevailed for decades, but it was not without skeptics. Some wondered why, if the immune system is the body’s main fighting force against pathogens, the brain would give up ready access to such a system of defense. Supporters of the theory responded that the blood-brain barrier prevents the entry of most pathogens into the brain, so the brain has no need to accommodate the immune system, especially if it could cause problems by being there—doing battle with neurons, for instance. The skeptics pointed out that several viruses, as well as some bacteria and parasites, can access the brain. And far from ignoring these transgressions, the immune system responds to them, rushing to the brain to manage the invading agent. Perhaps the scarcity of pathogens in the brain is not because the blood-brain barrier is so effective at filtering them out but because the immune system is so efficient at fighting them. Indeed, studies have shown that immunosuppressed patients suffer complications that often affect the CNS.
Rewriting the Textbooks
Eventually such arguments and a growing appreciation of the immune system’s role in supporting damaged bodily tissues prompted researchers to reexamine its role in the CNS. When they took a closer look at the CNS in rats and mice with spinal cord injuries, they found it overrun with infiltrating immune cells. In experiments carried out in the late 1990s, Michal Schwartz of the Weizmann Institute of Science in Rehovot, Israel, showed that eliminating immune cells after injury to the CNS worsens neuron loss and brain function, whereas boosting the immune response improves neuron survival. More recently, studies led by Stanley Appel of Houston Methodist Hospital and Mathew Blurton-Jones of the University of California, Irvine, have found that amyotrophic lateral sclerosis and Alzheimer’s disease develop more severely and rapidly in mice engineered to lack adaptive immunity than in normal mice. Restoring adaptive immunity slows the progression of such diseases. These results indicate that immune cells help neurons rather than only hurting them, as was previously supposed.
At first glance, the immune system’s intervention to protect the injured CNS does not make sense. When the CNS sustains trauma, the immune system mounts an inflammatory response, releasing toxic substances to eliminate pathogens and, in some cases, to remove damaged cells, thereby restoring equilibrium. The inflammatory response is a blunt instrument, however, taking out some of the good guys along with the bad. In other tissues, such collateral damage is tolerable because the tissues regenerate readily. But CNS tissue is limited in its ability to grow back, which means that damage from the immune response is typically permanent. Given the potential for immune activity to wreak havoc in the brain, the costs of intervention could often outweigh the benefits. But maybe the immune response observed after CNS injury is simply an extension of the immune response that aids brain function under normal conditions.
Studies from the past few decades support this notion. My collaboration with Hagit Cohen of Ben-Gurion University of the Negev in Israel and Schwartz revealed that mice that experience stressful stimuli, such as exposure to the smell of their natural predators, develop an immediate stress response—in this case, hiding in a maze rather than exploring it. In 90 percent of cases, the stress response disappears within hours or days. But for the other 10 percent, the response persists for days to weeks. Mice in the latter group can thus serve as an animal model for post-traumatic stress disorder (PTSD). Interestingly, in mice lacking adaptive immunity, the incidence of PTSD is increased severalfold compared with that among mice with a normal immune system. These results provided the first indication that the immune system supports the brain not only during infections and injuries but also during psychological stress. Moreover, some evidence links the immune system to PTSD in humans.
Though not as nerve-racking as exposure to a predator, tasks that require learning are also stressful. Think of preparing for an exam or even cooking a new recipe. Could an inability to deal with stress hinder the learning process itself? To test this hypothesis, my colleagues and I compared the performance of mice lacking adaptive immunity with that of a control group in various behavioral tests. We found that mice without adaptive immunity, unlike the controls, performed poorly in tasks requiring spatial learning and memory, such as figuring out the location of a platform hidden in a large pool of water. We have since shown that mice lacking adaptive immunity exhibit not only impaired spatial learning behavior but also compromised social behavior, preferring to spend their time with an inanimate object rather than another mouse.
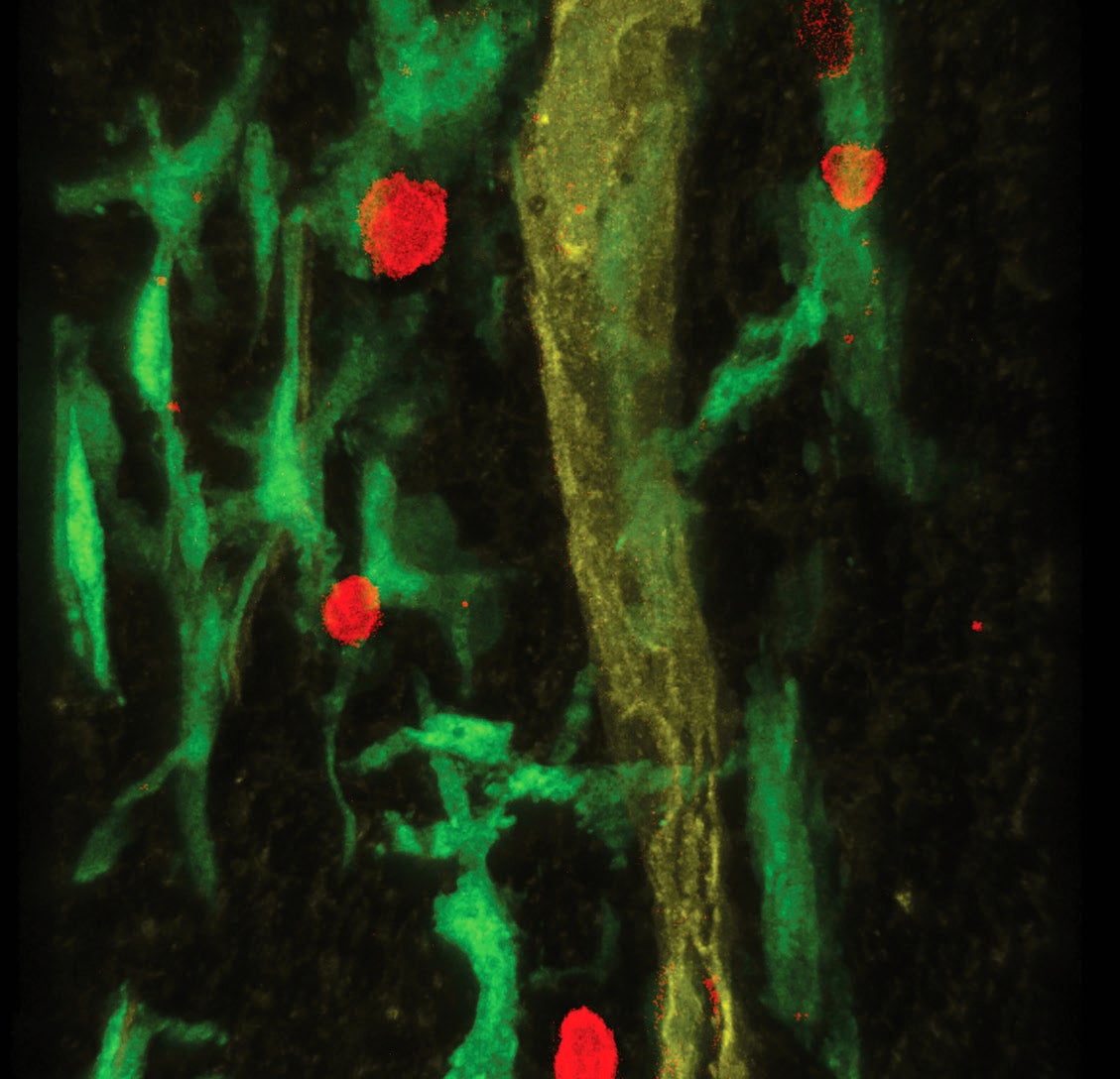
As evidence that the immune system plays important roles in different brain functions has accumulated, new unknowns have emerged. How the immune system exerts its influence in the CNS is one. After all, apart from microglia, no immune cells are present within the parenchyma of healthy individuals. Clues have come from proteins called cytokines, which are made by immune cells and influence the behavior of other cells. Cytokines released by peripheral immune cells can affect the brain. They presumably gain entrance through brain areas that lack the regular blood-brain barrier and could directly impact the brain through the vagus nerve, which runs from the brain to the abdomen. The available evidence suggests that the immune cells within the meninges—the membranes that surround the brain—are also the source of the cytokines that may affect brain function. How these immune cells enter the meninges, how they circulate there and how they produce their cytokines have become subjects of intensive research.
My colleagues and I made an intriguing discovery that bears on these questions. It has to do with how the body gets rid of toxins and waste. The tissues in the body contain two types of vessels. Just as a house has two types of pipes that serve it, one for water and the other for sewage, our tissues have the blood vessels that carry oxygen and nutrients to them and the lymphatic vessels that remove toxins and other waste materials that the tissues produce. The lymphatic vessels also ferry antigens—substances capable of inducing an immune response—from the tissues into tissue-draining lymph nodes, where they are presented to immune cells to be inspected for information on the draining tissue. On detecting a problem, such as injury or infection in the tissue, the immune cells are activated and migrate to the affected tissue to try to resolve the problem.
Because of the enduring belief that the healthy brain is disconnected from the immune system and because the parenchyma does not contain lymphatic vessels, scientists long assumed that neither the brain nor the rest of the CNS is serviced by the lymphatic network. Yet this assumption presented a conundrum: Why would the brain not report to the immune system about potential problems that might be affecting it and that the immune system might help solve? And how does the immune system nonetheless receive information on brain infections? Furthermore, studies have found that brain injuries provoke a strong immune response in lymph nodes located outside the brain. How is that possible?
Fascinated by the immune activity in the meninges and its effects on brain function, my colleagues and I decided to take a closer look at those membranes. In doing so, we made a serendipitous discovery: it turns out they house lymphatic vessels. Several other research groups have since made similar findings in fish, mice, rats, nonhuman primates and humans. The results confirm earlier proposals for a link between the brain and lymph system that were made some 200 years ago but largely dismissed. These vessels represent a bona fide lymphatic network that drains the CNS, a missing link that can relay information about brain infections and injuries to the immune system.
The presence of both lymph vessels and immune cells in the meninges means researchers have needed to rethink the exact function of these membranes. The traditional explanation holds that they simply carry the cerebrospinal fluid, which buoys the brain. But considering how densely packed the brain’s constituent cells are and how sensitive its neurons are when they fire their electrical signals, perhaps moving all of the brain’s immune activity to its meningeal borders was evolution’s solution to the problem of allowing the immune system to serve the entire CNS without interfering with neuron function.
The discovery of the brain’s lymphatic vessels revealed how the immune system receives information about tissue damage in the CNS. For insights into how the meningeal immune cells actually communicate with the parenchyma and affect it from afar, however, we have to turn to another branch of the brain’s waste-removal system. In addition to the lymphatic network that we discovered, the CNS also has a network of channels in the parenchyma through which the cerebrospinal fluid gets access to the brain. Maiken Nedergaard of the University of Rochester has dubbed this network the glymphatic system. The fluid enters the parenchyma through spaces surrounding the arteries that pipe into the brain from the meninges and washes through the tissues until it is re-collected in the spaces surrounding the veins and then returned to the pool of cerebrospinal fluid in the meninges. This flow of fluid presumably carries immune molecules such as cytokines from the meninges into the parenchyma, where they can exert their influence.
Studies of cytokines have illuminated how they modulate behavior. For example, Robert Dantzer, now at the University of Texas MD Anderson Cancer Center, and Keith Kelley, professor emeritus at the University of Illinois at Urbana-Champaign, determined that interleukin-1 beta initiates sickness behavior, the name given to the constellation of behaviors people typically exhibit when ill, such as sleeping excessively, eating less and withdrawing from social contact. And my own team has shown that interferon gamma, a cytokine produced by meningeal T cells, interacts with neurons in the brain’s prefrontal cortex, which, among its other functions, is involved in social behavior. Surprisingly, this cytokine exerts its influence not via the brain’s resident immune cells (the microglia) but rather through those neurons that control the circuits associated with social behavior. In fact, the cytokines are essential for proper functioning of these circuits: in the absence of T cells or their interferon gamma, these neurons fail to regulate the circuits correctly, and circuit hyperactivity ensues—a disturbance linked to social deficits. Thus, a cytokine produced by immune cells in the meninges can change the activity of neurons, thereby altering the function of the circuit and changing the underlying behavior.
Interferon gamma is not the only immune molecule that affects brain function. Mario de Bono of the Institute of Science and Technology Austria and his colleagues have shown that another cytokine, IL-17, activates sensory neurons in the roundworm Caenorhabditis elegans and changes the creature’s oxygen-sensing behavior. And work in mice by Gloria Choi of the Massachusetts Institute of Technology and her collaborators has demonstrated that IL-17 can interact with neurons in the brain’s cortex and alter behaviors related to autism spectrum disorder.
Another Sense Organ?
One might wonder why an organ as powerful as the brain needs to be controlled or supported by the immune system to function properly. I have developed a hypothesis for why the two systems are so closely linked. We have five established senses—smell, touch, taste, sight and hearing. The sense of position and movement, or proprioception, is often referred to as the sixth sense. These senses report to the brain about our external and internal environments, providing a basis on which the brain can compute the activity needed for self-preservation. Microorganisms abound in these environments, and the ability to sense them—and defend against them when needed—is central to survival. Our immune system excels at exactly that, with innate immunity’s ability to generally recognize patterns and types of invaders and adaptive immunity’s talent for recognizing specific invaders. I propose that the defining role of the immune system is to detect microorganisms and inform the brain about them. If, as I suspect, the immune response is hardwired into the brain, that would make it a seventh sense.
There are ways to test this hypothesis. Because the brain’s circuits are all interconnected, interference with one circuit tends to affect others as well. For instance, food tastes different when our sense of smell is impaired. Evidence that interference with immune input disturbs other circuits would support the idea that the immune response is a hardwired seventh sense. One possible example comes from sickness behavior. Perhaps an overwhelming input of signals from the seventh sense informing the brain of pathogenic infection spills over and disrupts the circuits that modulate sleepiness, hunger, and so on during illness, leading to this characteristic set of behavioral changes that develop in affected individuals. Alternatively, the microorganism information relayed to the brain by the immune sensory system may prompt the brain to initiate sickness behavior as a means of protecting the sick individual by minimizing exposure to other pathogens and conserving energy.
Our knowledge of the relation between the brain and the immune system is still in its infancy. We should not be surprised if new discoveries in this field over the next 10 or 20 years reveal the two systems in a completely different light. I hope, though, that the fundamental understanding we possess today will be enriched by the results of such research rather than overturned altogether. One research priority will be mapping how the immune components and neural circuits connect, interact and interdepend in health and disease. Knowing those relations will allow investigators to target immune signaling in their treatment of neurological and mental disorders.
The immune system is an easier drug target than the CNS, and it is plausible that one day repair of the immune system through gene therapy or even the replacement of a flawed immune system via bone marrow transplantation will be a viable means of treating brain disorders. Given the myriad immune alterations in these disorders, research on neuroimmune interactions will probably continue for decades to come and gradually reveal to us even deeper mysteries of the brain.