Salmon are so elemental to Indigenous peoples who live along North America’s northwestern coast that for generations several nations have called themselves the “Salmon People.” But when settlers came, their forms of agricultural and urban development devastated the mighty fish. The new inhabitants cut down streamside vegetation that once slowed and absorbed rains, causing floods. They straightened curvy creeks to try to speed floodwater off the land and armored the sides to prevent erosion, but the faster flow gouged the riverbed. Later, urban planners and engineers funneled streams into buried pipes so they could build more city on top, disconnecting waterways from soil, plants and animals. The cumulative impact of these injuries led to flash floods, unstable banks, heavy pollution and waning life. The hallowed salmon all but disappeared.
Across North America and the world, cities have bulldozed their waterways into submission. Seattle was as guilty as any until 1999, when the U.S. Department of the Interior listed Chinook salmon as threatened under the Endangered Species Act. That legally obligated the city to help the salmon when undertaking any new capital project that would affect the fish. Engineers trying to improve Seattle’s ailing streams began to reintroduce some curves, and insert boulders and tree trunks, to create more natural habitat, yet by and large, salmon did not return. Flooding also remained a hazard because rain rushed off the hardened cityscape into the still mostly inflexible channels, which overflowed.
In 2004 biologist Katherine Lynch was sitting through yet another meeting on how to solve these problems—this one held by her employer, Seattle Public Utilities—when she had an epiphany. Maybe restoration projects were failing because they were overlooking a little-known feature damaged by urbanization: the stream’s “gut.”
A stream is a system. It includes not just the water coursing between the banks but the earth, life and water around and under it. Lynch had been tracking discoveries about a layer of wet sediment, small stones and tiny creatures just below the streambed called the hyporheic zone—a term from the Greek hypo, meaning “under,” and rheos, meaning “flow.” Stream water filters down into this dynamic layer, mixing with the groundwater pushing up. Water in the hyporheic zone flows downstream like the surface water above it but orders of magnitude more slowly.
For a large river the hyporheic zone can be dozens of feet deep and can extend up to a mile laterally beyond the banks. It keeps the waterway healthy by regulating critical physical, biological and chemical processes, including riverbed aeration, water oxygenation, temperature moderation, pollution cleanup and food creation. Some biologists compare the hyporheic zone to the human gut, complete with a microbiome. Others call it the liver of the river.
A healthy hyporheic zone is full of life. Crustaceans, worms and aquatic insects constantly move between the zone and surface flow. Nematodes, copepods, rotifers and tardigrades also dig up and down, creating spaces for water to mix underground. Microbes proliferate throughout the zone. Water welling up from below brings oxygen to salmon eggs laid in the riverbed. Lynch realized that few people trying to restore Seattle’s streams were thinking about the hyporheic zone, or that the channelizing of streams scours it away, or that putting streams in pipes disconnects the zone from the stream water above.
The meeting concerned Seattle’s Thornton Creek, which originally wove through rich lowland rain forest, draining an 11.6-square-mile watershed before emptying into Lake Washington. Developers had straightened it and armored it with rocks or concrete, squeezing it into channels only a few feet wide in some places. Its 15-mile course ran along a highway for a while and carved through hundreds of backyards. Some houses were so close to the narrowed stream that their decks overhung the water. Thornton had a reputation as the most degraded creek in the city—and as a dangerous one: it flooded a major road nearly every year, blocking access to schools, a community center, hospitals, businesses and bus routes. At times homes and a high school flanking the creek also flooded.
Talk at the meeting centered on the best practices of the time: reconnecting the stream with some of its floodplains by reclaiming adjacent property, removing armoring and reintroducing native plants along the banks. Lynch boldly told the group the project should go further: rebuild the missing hyporheic zone. That would mean reclaiming space under the stream, filling it with sand and gravel and potentially bringing back the zone’s tiny inhabitants.
As far as Lynch knew, no one had tried to rebuild a missing hyporheic zone in an urban stream. She hoped that restoring the stream’s gut would help Thornton Creek better maintain itself, reducing the need for ongoing, expensive human assistance. She also argued that if the revolutionary approach succeeded, it would set a new standard for urban stream restoration at a time when flooding around the world was routinely costing human lives and billions of dollars in damages. Cities everywhere had confined and subsumed many thousands of streams, erasing them from public memory. One study found that Philadelphia had buried 73 percent of its streams. Another study counted 66 percent buried in Baltimore. Globally many streams that remained on the surface were sick or dying. Restoring Thornton Creek’s hyporheic zone could create a blueprint for enhancing biodiversity while also reducing urban flooding and drought.
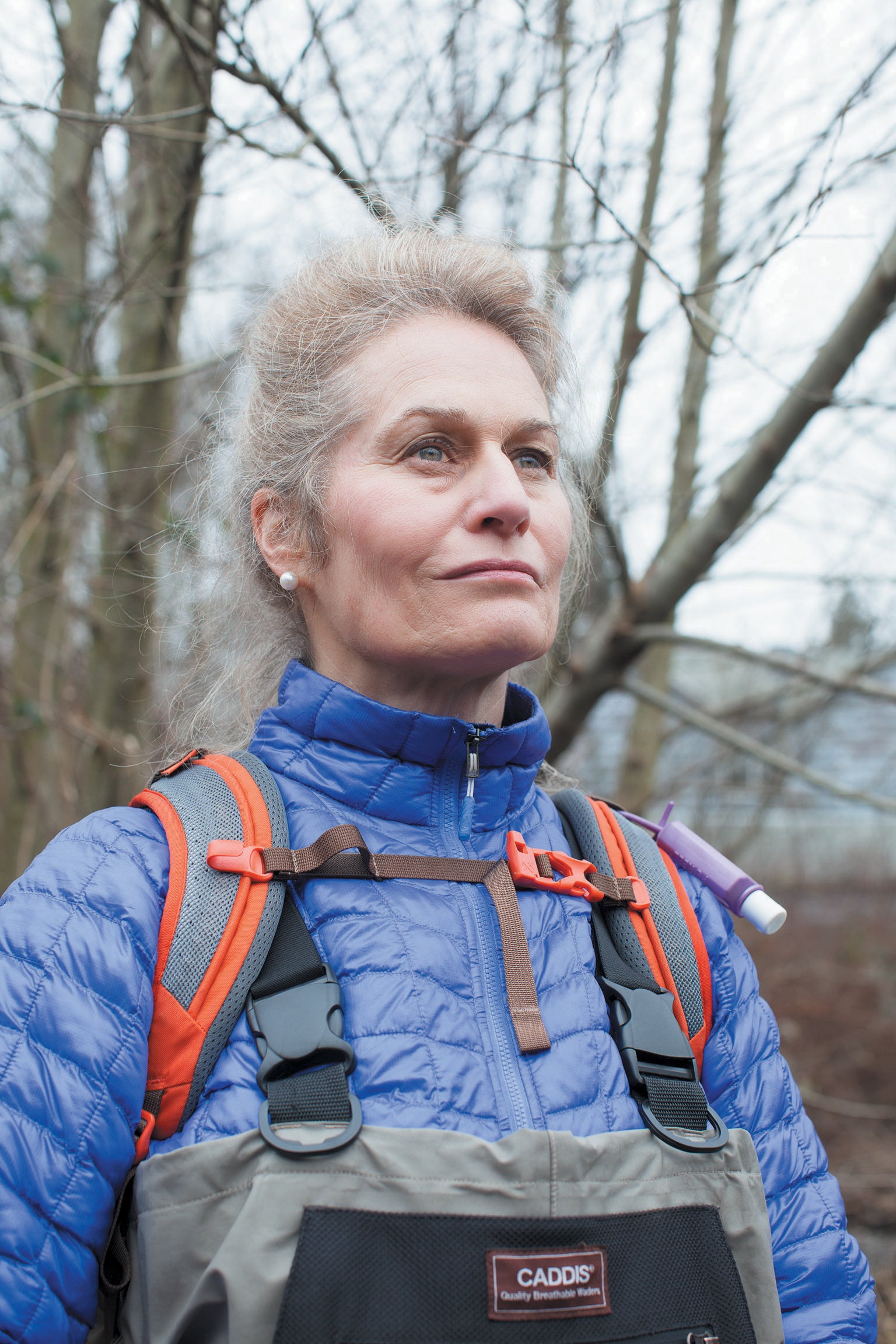
Fellow scientists at the meeting were enthusiastic about Lynch’s radical proposal. But at subsequent meetings she quickly encountered a basic hurdle among the other decision-makers. “People,” she says, “had no idea what I was talking about.”
Life in the Zone
The hyporheic zone is a vibrant place. Its water chemistry, temperature and life-forms differ from those in the stream above and the groundwater below. These kinds of in-between ecosystems are called ecotones—liminal spaces that can harbor great biodiversity because species from neighboring environments mingle there, along with microbes and other critters that reside only in that space.
The tiny beings in the hyporheic zone function as ecosystem engineers, metabolizing inorganic compounds into food for plants and bugs. They move organic matter and nutrients between the zone and riverbed sediments and play a pivotal role in nitrogen, phosphorus and carbon cycles. The hyporheic also helps to regulate a stream’s temperature, bringing in comparatively cooler underground water in the hot summer and warmer underground water in the cold winter.
Scientists have shown how wide and deep a hyporheic zone can reach by mapping aquatic insects and fish embryos found in soil beyond a waterway’s banks. For an urban creek such as Thornton, that lateral reach might extend 30 feet from the stream channel. The depth might be three feet below the streambed.
Straightening a stream and building over its floodplain can destroy the hyporheic zone. It also compounds problems: Rain that falls on pavement and rooftops cannot soak into soil and instead races off these hard surfaces, picking up fine dirt and pollutants as it rushes into the stream. These flows, which ecologists call “flashy,” create a firehose effect that scours the riverbed and the hyporheic material underneath it, laid down over centuries. Eventually what remains is the impermeable underbelly, such as shale or granite. And a straight, armored river channel often cannot contain the flashy runoff; water overflows the banks, flooding the area.
Thriving floodplains absorb potential floodwater. They also slow water, dissipating its energy and reducing erosion. Slow water more readily sinks underground, where some of it will return to the stream over time via the hyporheic, supplying water in dry times. Natural streams with a stable hyporheic have a more balanced flow between winter and summer, helping to maintain water in streams year-round, even in drought-prone areas.
All these processes enable a stream to maintain itself. If the hyporheic zone is stripped away, a stream’s biological gut disappears, and the waterway has little hope of staying healthy—akin to when humans develop serious digestive tract issues because their gut microbiome has been distressed.
Hyporheic Rebuild
Lynch first learned about the hyporheic zone in 2000 at the University of Washington, but she did not appreciate how extensive the zone is until a 2004 field trip into a forest with geomorphologist and visiting lecturer Tim Abbe. She was amazed when they stopped walking and he pointed out that the ground they were on overlaid a hyporheic zone for a nearby stream. “I’m looking around at trees and ferns,” she recalls, “thinking, How is that possible?”
Born and raised in Nova Scotia, Lynch had moved to the U.S. Pacific Northwest and ended up working for Seattle Public Utilities, focusing on stream restoration. The two stretches of Thornton Creek slated for revitalization and discussed at the 2004 meeting were called Confluence and Kingfisher. They totaled 1,600 feet in length. The team chose these spans because they were originally floodplains, and allowing overflow there could greatly reduce problematic flooding along the stream’s longer route. The Seattle Parks Department had already been buying out willing homeowners whose houses flooded along those stretches—five at Confluence and six at Kingfisher—so some of the creek’s stolen elbow room could be restored.
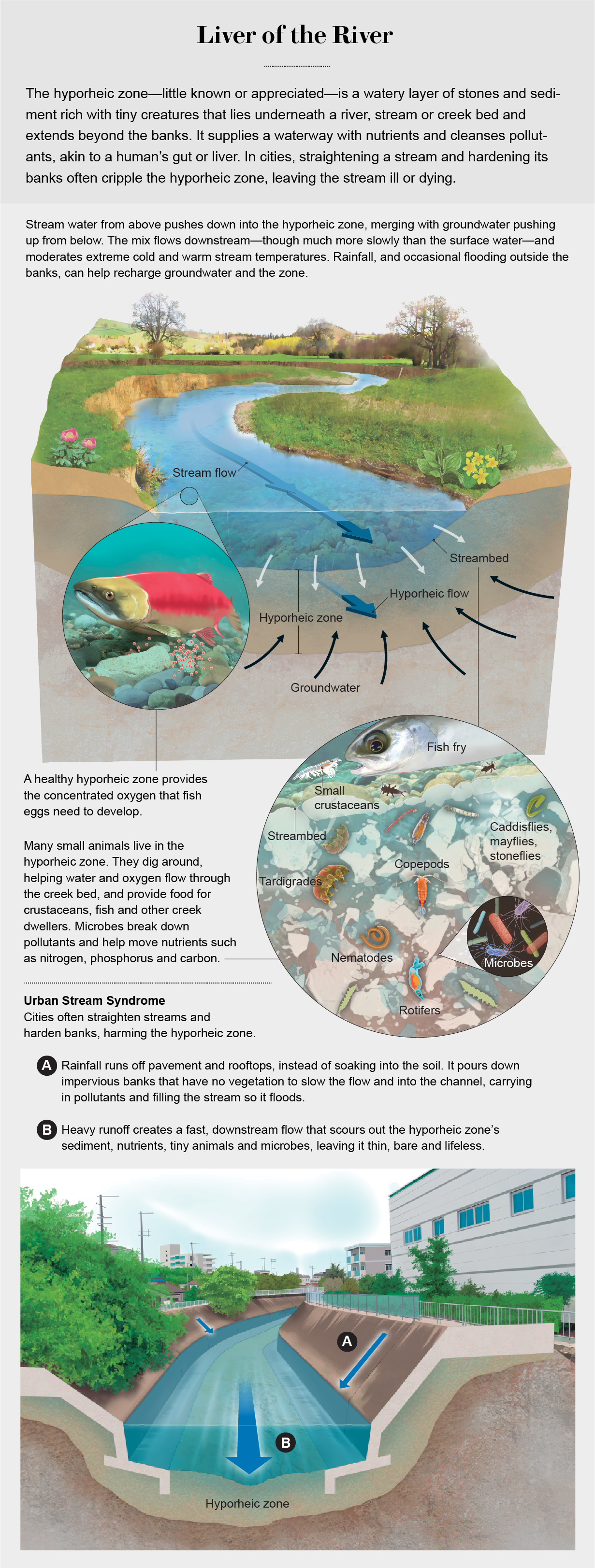
Lynch knew that getting decision-makers to try something new would be hard. Urban stream restorations have big price tags and high stakes—namely, ensuring that people’s properties do not flood. By 2007, after much discussion, the design plans included hyporheic restoration—although it was not approved as a formal part of the project for another seven years. That time line is typical of city projects, Lynch says, which require funding; coordination among landowners, community groups and multiple agencies; and assessments of social justice and equality.
Lynch’s supervisor asked that the work include stream monitoring so scientists could provide data to inform subsequent projects. Paul Bakke, then a geomorphologist at the U.S. Fish and Wildlife Service, did baseline measurements, which confirmed that Thornton Creek’s hyporheic zone had been almost completely scraped away. The utility hired Seattle-based Natural Systems Design, a science and engineering firm that restores waterways. Lynch teamed Bakke with the firm’s lead engineer, Mike Hrachovec, to create the innovative design.
The restoration was personal for Bakke, who had grown up in the 1960s and 1970s along Thornton Creek, fishing for cutthroat trout and playing with water skeeters. Just before he entered high school, the city issued permits for condos along the creek’s edge, cutting off his access to the water. “These old haunts that I really loved, that were my sort of wilderness ... were suddenly not just blocked but being paved over,” he says. “It was very upsetting.”
Hrachovec also frequented streams in his youth, in South Dakota’s Black Hills. Nevertheless, when Lynch paired them up to redesign Thornton Creek, the two men found collaboration rough going. In one battle, Bakke wanted to put larger gravel on the streambed so water could move more easily into the hyporheic. Otherwise, he feared, urban dust washing into the creek could plug up the downward flow. Hrachovec worried that large gravel might convey too much water underground, drying out the surface stream in summer and killing fish. This kind of uncertainty is one reason it can be hard to get a city to try something new.
Stream shape, gradient, water speed and debris also influence flow into and out of the hyporheic zone. To sort things out, the team ran tests using computer simulations and in a large sandbox, modeling stream dynamics and trying different rock aggregates, curves and wood placement to drive water underground. Satisfied at last, and with other city requirements in place, Seattle put out a call for bids in early 2014. Then, in May 2014, just before construction was due to begin, the Seattle Public Utilities project manager raised budget concerns because another project was running over. “In front of my eyes,” Lynch recounts with incredulity, “he says, ‘What’s this hyporheic thing?’ And he just cut it.”
Lynch told the manager how crucial the zone was and argued that the hyporheic elements accounted for only $300,000 of the two sites’ combined $10.5-million budget. She told him the investment—for excavation and materials such as boulders, gravel and finer sediment—was likely to pay off quickly. Her team had determined that rebuilding the zone would reduce the need to spend $1 million a year on average dredging sediment from a nearby stormwater pond built to absorb heavy runoff.
She also reminded the manager that the monitoring would provide lessons on how to reconstruct urban streams to something closer to their full complexity, making Seattle a leader in this work worldwide. In the end, they negotiated. Lynch was able to keep the Confluence hyporheic restoration intact; the Kingfisher reach was shortened by 25 percent.
In summer 2014 the bulldozers moved in. Hrachovec and his team scooped out generous curves in the spaces reclaimed from the houses, in spots widening the creek from four or eight feet to 25 or 30 feet. To bring the creek bed to its former elevation and reintroduce material that would hold the hyporheic zone, they layered in sediment and gravel nearly eight feet deep. Hrachovec and Bakke inserted logs of different sizes into the water at precise angles—some partly buried, some crisscrossing the streambed—creating tiny waterfalls, plunge pools and pockets of nearly still water that create hydraulic pressure that can force water down into the zone. These meticulously placed logs and boulders, known as “hyporheic structures,” also create eddies and pockets of slow water that provide safe havens for juvenile fish and bugs—all meant to emulate features of a natural stream.
The Kingfisher reconstruction was finished by that fall and the Confluence by spring 2015. The creek’s flow slowed, so sediment dropped out of the water column and began refining the stream’s shape and bed. That action also reduced what had been rapid downstream sediment accumulation that the city had removed regularly at great expense. Over the next five years gravel and silt gradually built up behind the wood barriers, creating gentler grades.
The monitoring allowed Bakke and Hrachovec to track water flow by sensing temperature and following tracers. They confirmed that water was indeed moving down and through the hyporheic zone. In a 2020 paper, they reported that water was mixing there at 89 times the preconstruction rate. Data analysis proved the stream was working as Bakke and Hrachovec—and nature—intended.
But was that flow also supporting life and reducing pollution?
Bugging the Creek
Restoring a stream’s natural shape can encourage displaced plants and animals to move back in. In many cases, however, only some species return. And because the gravel and sand the team installed were sterile territory, Bakke thought they might need a biological jumpstart.
If a species is missing from an ecosystem, our instinct is simply to reintroduce it. But ecologists are painfully aware of cautionary tales such as stocking a desirable trout that inadvertently brings pathogens along with it. Even bringing back a native plant can shake up a system that has adjusted to its absence.
Kate Macneale, an environmental scientist for King County, where Seattle is located, understands this lesson. She monitors insects as a measure of stream health, rating them on what she calls the “bug score.” Macneale had found a clear correlation between urbanization and lower bug scores; some species, she figured, were too sensitive to survive.
A few years ago an experience made her rethink that conclusion. Vandals had destroyed an experiment she had set up in Seattle’s Longfellow Creek, which released what had been captive bugs into the “wild” of the urban stream. Two years later she was sampling fish there and found one of the bugs, a caddisfly, in a fish’s gut. Caddisflies live only for a matter of weeks, so it could not have been an individual from the unintended release: it must have been a “grandkid of that individual,” she says. “I couldn’t believe it.”
Macneale realized that some insects in reconstructed streams might be missing not because they cannot hack the conditions but because there were no nearby insects around to recolonize the water. The idea that life will return to restored creeks relies on critters migrating from healthy upstream habitats. But with Longfellow Creek, Macneale says, the headwater “is literally a Home Depot parking lot.” If organisms are to recolonize restored streams, she says, “we may need to help them out.”
With that insight, she got permission from King County to seed four creeks with caddisflies, mayflies, stoneflies and other species. Some of them survived. In 2019 the Thornton Creek team tried another groundbreaking move: inoculating the engineered hyporheic zone with life. In keeping with the human gut analogy, the procedure is somewhat like administering probiotics, or even a fecal transplant, to a person to restore their gut microbiome.
Enter Sarah Morley, a stream ecologist, and Linda Rhodes, a microbiologist, both with the National Oceanic and Atmospheric Administration. They harvested wild microbes and invertebrates in small baskets placed in the healthier Cedar River watershed nearby. They took a few baskets back to the laboratory to document captured species, and they buried the others in Thornton Creek’s restored hyporheic zones.
Invertebrates and microbes quickly colonized the areas. But even though the number of individuals was high, the biodiversity was relatively low. According to the duo’s 2021 paper in Water, a few of the new species proliferated, but most of the other species were similar to those in unrestored sections of the creek.
Morley and Rhodes are considering why more of their introduced species did not make it. Because this science is so new, they have not ruled out any potential explanations. The donor stream may be too different, or the restored area too small, or water quality too poor. They might have inoculated the hyporheic too soon, before small vegetation needed by some critters could grow. And yet in the guts of some trout, Lynch found aquatic insects that had not been seen in Thornton Creek for at least 20 years. “The fish are better at sampling than we are,” she says. The scientists are now conducting another study with more sensitive monitoring.
Still, Morley and Rhodes did find that the microbes that began living in the restored stream sections were much more active than those in nearby unrestored sections, indicating they were “getting goosed to do something,” Rhodes says—maybe build biofilms and biomass, clean pollutants or break down organic material. The restored sections had seven times more hyporheic crustaceans, worms and insects, as well as much greater overall species diversity.
Tracking Chemicals
The final question about the Thornton Creek restoration was whether it was cleaning pollution that pours in with runoff during storms, from lawn fertilizer to urban wastes. Lynch had to search for three years to find a chemist who would conduct the research. “All of them said it could not be done,” she recalls. They said it was too difficult to track how long water stayed in the hyporheic zone and to measure whether chemicals were removed while the water spent time underground.
Lynch eventually reached Skuyler Herzog, then an engineer at the Colorado School of Mines, who specializes in the hyporheic zone. “He took the next plane out here,” Lynch recounts with glee. After years of studying the hyporheic zone academically, he was thrilled to conduct tests on a real restoration. Lynch recruited University of Washington chemist Edward Kolodziej to help.
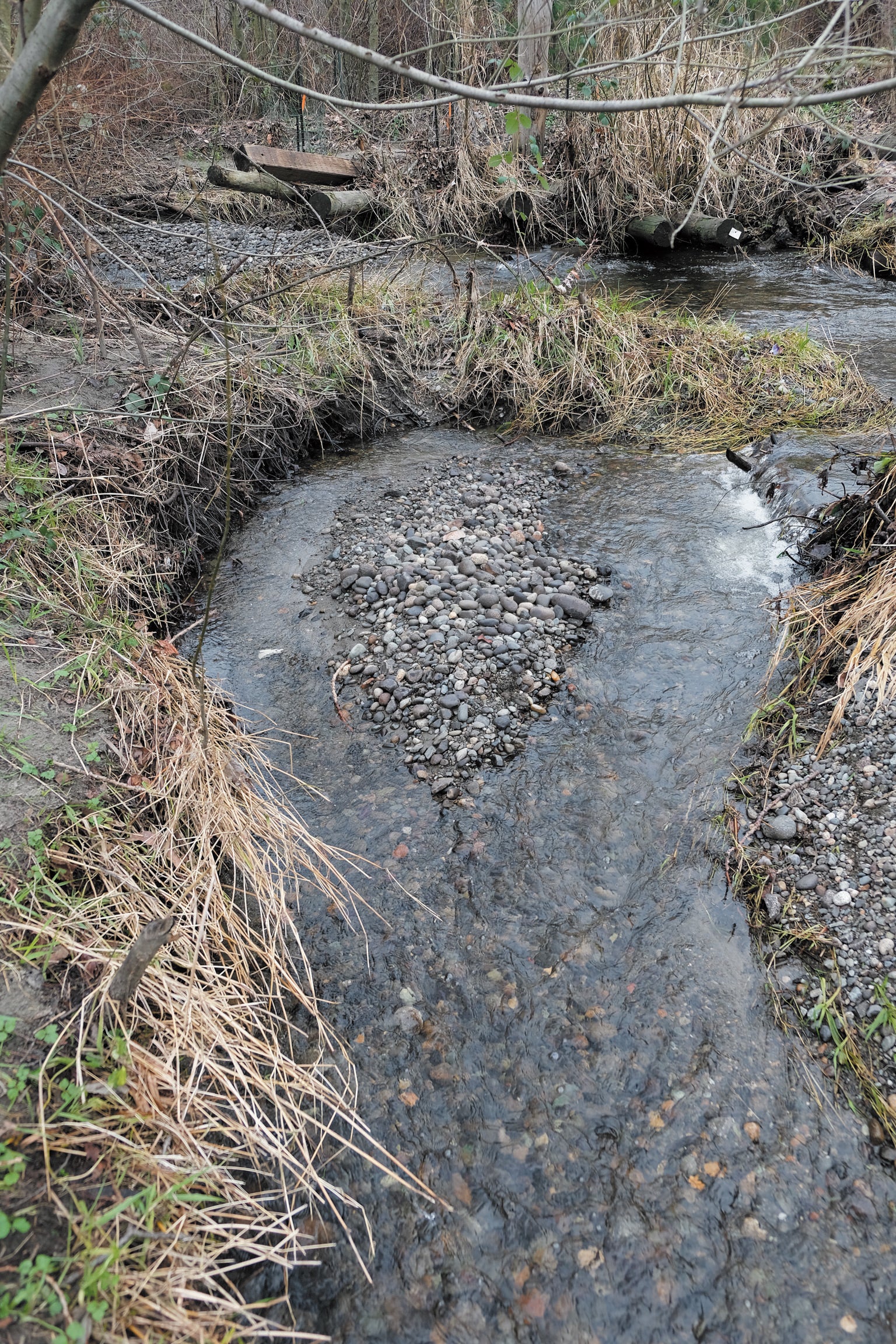
The team sent tracer dyes into an engineered plunge pool that pushed water into the hyporheic. They then monitored exit points seven and 15 feet downstream to determine how long a “packet” of water stayed under before rejoining surface flow; water stayed under for 30 minutes to three hours or more. They also collected water samples from the stream and used mass spectrometry to measure different pollutants from storm runoff. They counted nearly 1,900.
The scientists sampled water packets before they entered the stretches of hyporheic and after they emerged and compared them with water flowing downstream above the stretches. The surface flow reduced the concentration of about 17 percent of the chemicals by at least half. The seven-foot stretch of the hyporheic reduced the concentration of 59 percent of the chemicals by at least half, and the 15-foot stretch reduced the concentration of 78 percent of the chemicals by at least half. Because water spent so little time in those short hyporheic stretches, the team thinks the pollutants mostly got stuck on sediments or biofilms rather than being broken down immediately by microbes, although that decomposition is common over longer time periods.
Hrachovec says it is “jaw-dropping” that such short hyporheic spans were able to reduce so much pollution. He adds it was “astounding to contemplate how much good we could do if we had this more available.”
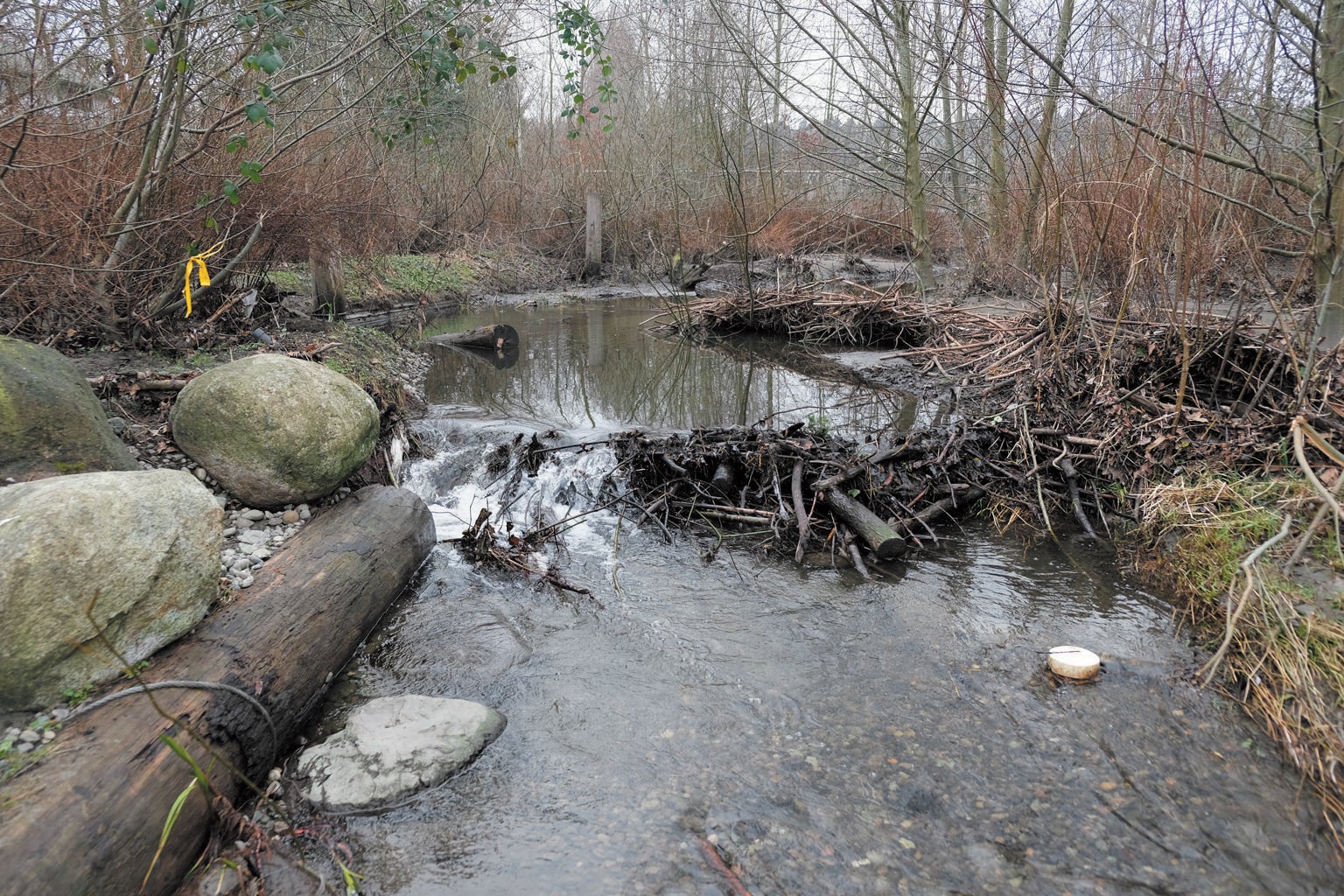
A New Standard?
The Thornton Creek findings are encouraging. The neighborhoods around the creek have not flooded since the restorations were finished in 2015, even during large storms. The stream’s temperature and flow are more consistent year-round. The city needs to dredge less often, saving money, and neighbors love spending time in the expanded green space. Yet the work also reveals how complex nature’s systems are and how difficult it can be to restore them once damaged. As cities and agencies increasingly turn to more nature-based solutions, the Thornton Creek lessons can help experts understand which steps work and which need improvement.
Success has helped Lynch convince Seattle Public Utilities and other city decision-makers of the importance of a stream’s gut. Hyporheic restoration has become a formal part of the utilities’ creek projects—not guaranteed but routinely considered. Taylor Creek now has eight planned hyporheic reconstructions along a 1,200-foot stretch. Herzog is testing design improvements to increase water’s “residence time” in the hyporheic and is studying how much that increases cleansing. Plans to restore the north branch of Thornton Creek include hyporheic structures. Because of the zone’s power to reduce pollution, the city will probably include hyporheic structures in a restoration along Longfellow Creek, which contains a chemical from vehicle tire particles that Kolodziej has shown kills salmon; construction could begin by 2026.
Still, small restorations cannot fully compensate for insults to long streams and rivers. “Stormwater runoff, biodiversity, flooding—these are watershed-scale problems,” Bakke says. That is why reconstructions need to be distributed in many places along a stream or river. Abbe, the geomorphologist who inspired Lynch, is now at Natural Systems Design. He has planned and overseen 14 hyporheic restorations in five other Washington State counties. In 2019 Abbe was walking along a project on Poison Creek in Chelan County with Steve Kolk, an engineer with the U.S. Bureau of Reclamation, an agency infamous in ecology circles for building giant dams. As Abbe tells it, Kolk suddenly stopped walking and said, “Ultimately you’re talking about hundreds of thousands of these treatments to restore our watershed.” Abbe said, “Bingo.”
Finding space for more natural water flows in an established city might seem difficult, but buildings are replaced more often than people think, particularly when they flood regularly. Cities can reclaim that land, as Seattle did. Even small projects in key places can make a difference. By restoring the floodplains at Confluence and Kingfisher, Seattle has relieved troublesome flooding along Thornton Creek.
Most exciting for Lynch, the hyporheic innovations won the ultimate stamp of approval in the fall of 2018, when Chinook salmon swam in from Puget Sound and spawned in the creek’s restored hyporheic zones.
“That was just really emotional,” Lynch recalls. “We had done it. You can restore the hyporheic zone. You can restore natural processes to the extent that we are actually attracting salmon to the site to spawn.” If these two small restorations in an urban creek can help restart a functioning ecosystem, she says, “I think there really is hope for the future.”
Related
Engineering with Nature - sustainable solutions for a changing climate from S Solomon Leaping Frog Films on Vimeo.