The summer of 2021 was a glaring example of what disruptive weather will look like in a warming world. In mid-July, storms in western Germany and Belgium dropped up to eight inches of rain in two days. Floodwaters ripped buildings apart and propelled them through village streets. A week later a year’s worth of rain—more than two feet—fell in China’s Henan province in just three days. Hundreds of thousands of people fled rivers that had burst their banks. In the capital city of Zhengzhou, commuters posted videos showing passengers trapped inside flooding subway cars, straining their heads toward the ceiling to reach the last pocket of air above the quickly rising water. In mid-August a sharp kink in the jet stream brought torrential storms to Tennessee that dropped an incredible 17 inches of rain in just 24 hours; catastrophic flooding killed at least 20 people. None of these storm systems were hurricanes or tropical depressions.
Soon enough, though, Hurricane Ida swirled into the Gulf of Mexico, the ninth named tropical storm in the year’s busy North Atlantic season. On August 28 it was a Category 1 storm with sustained winds of 85 miles per hour. Less than 24 hours later Ida exploded to Category 4, whipped up at nearly twice the rate that the National Hurricane Center uses to define a rapidly intensifying storm. It hit the Louisiana coast with winds of 150 miles an hour, leaving more than a million people without power and more than 600,000 without water for days. Ida’s wrath continued into the Northeast, where it delivered a record-breaking 3.15 inches of rain in one hour in New York City. The storm killed at least 80 people and devastated a swath of communities in the eastern U.S.
What all these destructive events have in common is water vapor—lots of it. Water vapor—the gaseous form of H2O—is playing an outsized role in fueling destructive storms and accelerating climate change. As the oceans and atmosphere warm, additional water evaporates into the air. Warmer air, in turn, can hold more of that vapor before it condenses into cloud droplets that can create flooding rains. The amount of vapor in the atmosphere has increased about 4 percent globally just since the mid-1990s. That may not sound like much, but it is a big deal to the climate system. A juicier atmosphere provides extra energy and moisture for storms of all kinds, including summertime thunderstorms, nor’easters along the U.S. Eastern Seaboard, hurricanes and even snowstorms. Additional vapor helps tropical storms like Ida intensify faster, too, leaving precious little time for safety officials to warn people in the crosshairs.
Scientists have long anticipated that climate change would create more airborne vapor, fueling what might be called “vapor storms” that are unleashing more rain and snow than storms did only a few decades ago. Measurements confirm that heavy-precipitation events are hitting harder and occurring more often across the U.S. and the globe. Since the late 1980s about one third of U.S. property damage caused by flooding—$73 billion—has been attributed to increases in heavy precipitation.
In August 2017, for example, Hurricane Harvey dumped a mind-boggling five feet of rain in some Houston neighborhoods over the five days it dawdled in the region, leaving even well-weathered meteorologists speechless. At times the rainbands dropped an astounding six inches of precipitation per hour. One analysis concluded that the record-smashing rainfall was made three times more likely and 15 percent more intense by climate change, in particular the moisture-laden air that fed Harvey from the abnormally warm Gulf of Mexico.
Unlike most other atmospheric gases, water vapor is not evenly distributed around the globe. Vapor is abundant in the steamy tropical regions straddling the equator. From there, long tendrils of moisture can extend toward the cooler, drier poles along storm tracks, bathing mid- and high-latitude regions in bouts of intense, prolonged precipitation. These rivers of heat and moisture help to balance Earth’s atmospheric energy distribution—and they are creating strong vapor storms along their path.
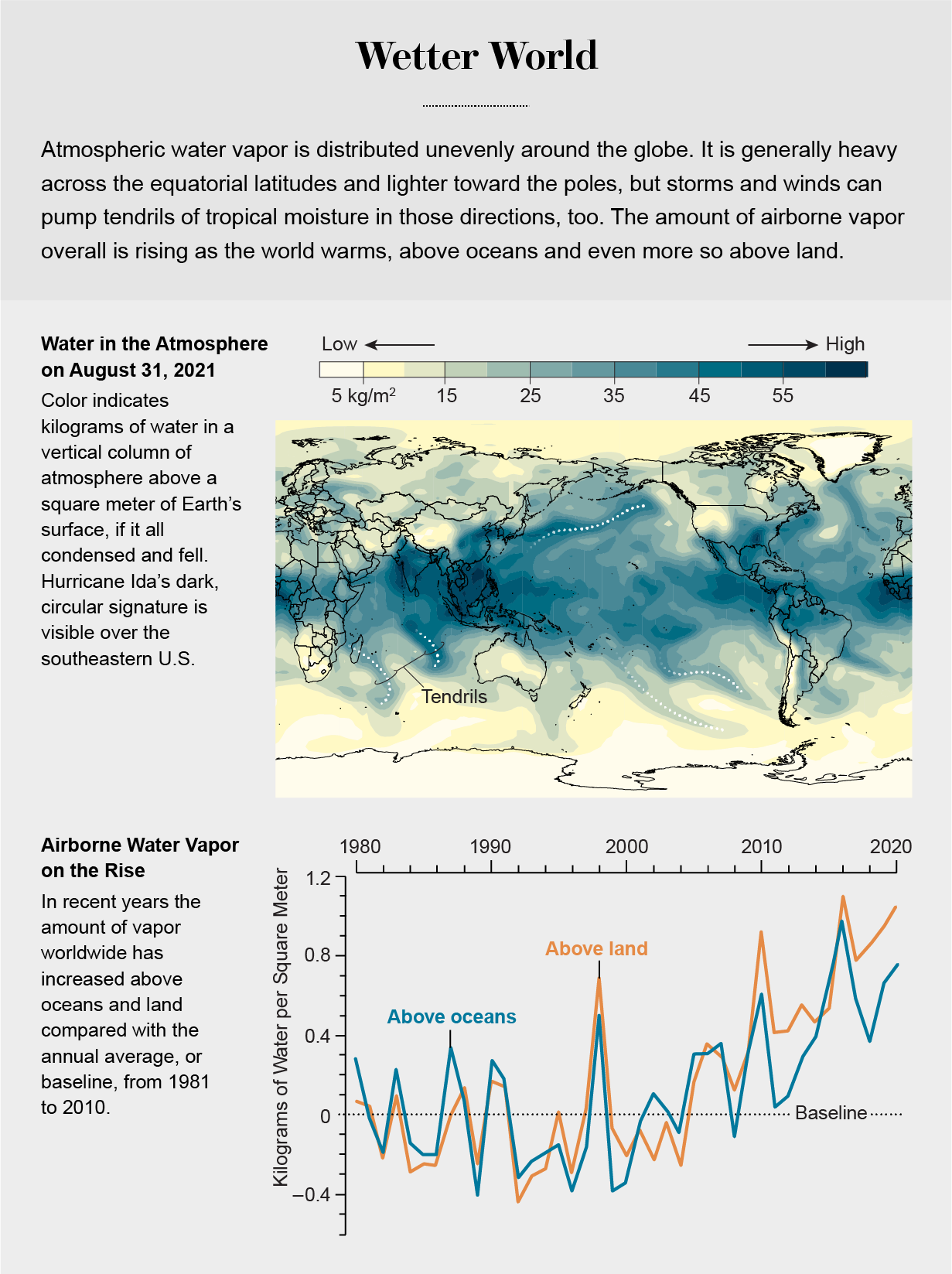
Energy Pump
When we sweat under a hot sun or set a pot to boil on our kitchen stove, we convert liquid water into water vapor. The necessary ingredient is heat. Similarly, heat in the climate system causes water in moist soil, plants, oceans, lakes and streams to evaporate into the air. The vapor carries with it a form of energy called latent heat. If the vapor later condenses back into liquid—forming a cloud or dew on a lawn—that heat is released into the atmosphere. The resulting bubble of warm air is lighter than the air around it, so it rises. Because temperatures are generally cooler at higher altitudes, the bubble can continue to rise and grow, all the while condensing additional water vapor into cloud droplets and releasing yet more latent heat. If you have flown in an airplane through a big, cauliflower-shaped cloud, you have felt the turbulence created by these towers of rising air.
Latent heat is the main fuel that powers hurricanes, thunderstorms and normal bouts of lousy weather. The energy contained in latent heat is substantial; in a typical hurricane, the amount of heat energy released in one day is more than 200 times the energy in all the electricity produced worldwide per day. A hurricane can release the explosive power of a 10-megaton nuclear bomb about every 20 minutes.
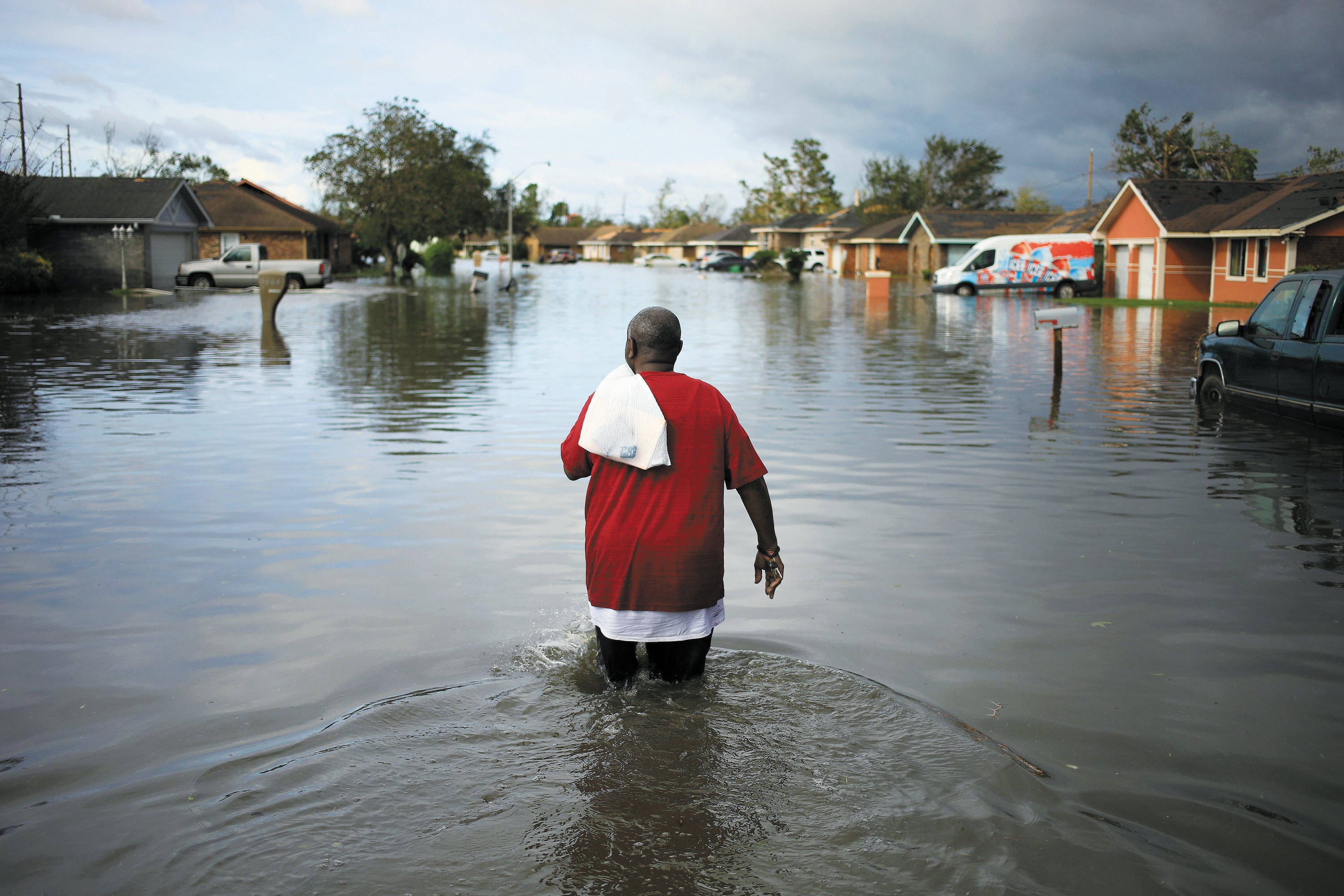
The most worrisome consequence of increasing atmospheric water vapor may be its role in the rapid intensification of tropical storms. Meteorologists say a storm has rapidly intensified when either the maximum wind speed increases by at least 30 knots (35 miles per hour) in a 24-hour period or the storm’s central atmospheric pressure drops at least 42 millibars in 24 hours. In the past 40 years the probability that a storm will rapidly intensify in any given year has quintupled. In 2020 alone, 10 Atlantic hurricanes did just that: Hanna, Laura, Sally, Teddy, Gamma, Delta, Epsilon, Zeta, Eta and Iota. In 2021 five of the six Atlantic hurricanes that formed as of mid-September underwent rapid intensification, including Ida and Nicholas. Recent studies agree with physical common sense: rapid intensification becomes increasingly likely as oceans warm, evaporating more water and delivering more latent heat to the atmosphere. Oceans absorb about 90 percent of the heat trapped by extra greenhouse gases we humans have emitted. That heat raises water temperatures both at the surface and deeper below; the warm water acts like a powerful battery that storms can draw energy from.
Increasing water vapor is not the only impact of climate change on tropical storms, however. Decreasing wind shear—the difference in speed or direction between winds closer to the ground and those high in the atmosphere—also favors storm development because the towers of rising air are less likely to be torn apart. Other variables now being studied include changes in the amount of dust and pollution particles in the air, as well as differences in atmospheric warming at lower and higher altitudes, which affect how fast those bubbles of warm air rise.
For more than two decades much of the tropical North Atlantic Ocean has been abnormally warm, creating excess evaporation that fuels strong hurricanes. Nontropical storms are gorging on the atmosphere’s extra vapor and energy, too, leading to more heavy-precipitation events and perhaps even heavier snowfalls.
Deadly Heat
The threat from increased water vapor extends beyond storms. It is also making summer nights intolerably steamy—more often and in more places.
Since the mid-1990s summer nighttime minimum temperatures over global land areas have been rising faster than daytime highs. That is because vapor is a greenhouse gas, and more of it means more warming: heat that would normally escape to space at night is instead trapped, preventing Earth’s surface from cooling. And unlike carbon dioxide, which spreads worldwide regardless of where it is emitted, vapor tends to stay local.
More vapor also makes hot nights perilous. Higher nighttime humidity prevents your sweat from evaporating—the body’s natural cooling system—leaving you to overheat and interfering with sleep. One measure of this discomfort is the heat index, which combines the effects of temperature and humidity to represent the stress one’s body really feels. An index above about 100 degrees Fahrenheit (38 degrees Celsius) is considered dangerous; prolonged exposure can be fatal, especially to the elderly and infants. Heat stresses livestock and pets, too, and animals in the wild are adapting by moving toward higher latitudes or higher elevation if they can. Without a period of nighttime cooling, heat can also build up in soils, killing some plants and insects while allowing other, warmth-loving species to flourish. According to “A Declaration on Climate Change and Health 2021,” published in August by a group of 32 health organizations, nighttime heat also heightens the risk of exposure to diseases carried by insects, threatening humans, animals and crops.
The danger posed by nighttime heat is growing not only for already hot tropical countries but also for those well north and south of the equator. Cities along the U.S. Gulf Coast have already exceeded the unsafe threshold many times. Warming in Houston has risen more than 3.5 degrees F (two degrees C) since 1970 because of the city’s proximity to the Gulf of Mexico and its relentlessly expanding development, which is augmenting the urban heat-island effect. In July 2020 Houston’s heat index topped 110 degrees F (43 degrees C), well beyond miserable.
If greenhouse gases continue to accumulate in the atmosphere, these conditions will soon become commonplace in many southern and midlatitude cities such as Atlanta and Washington, D.C. Before 2000 the U.S. capital experienced an average of one night every five years with a minimum temperature above 80 degrees F (27 degrees C). Since 2000 these nights have occurred about twice a year—a 10-fold increase in just 20 years.
Yet certain countries in the tropics will suffer, and are already suffering, the most. In May 2015 a severe heat wave, perhaps better termed a “steam wave,” hit India and Pakistan. Daytime heat indices exceeded 114.8 degrees F (46 degrees C) for several days, and the high humidity prevented nighttime cooling; more than 3,500 people succumbed to these stifling conditions. Add another half-degree of global warming and the number of people threatened by extreme heat will double to about 500 million worldwide.
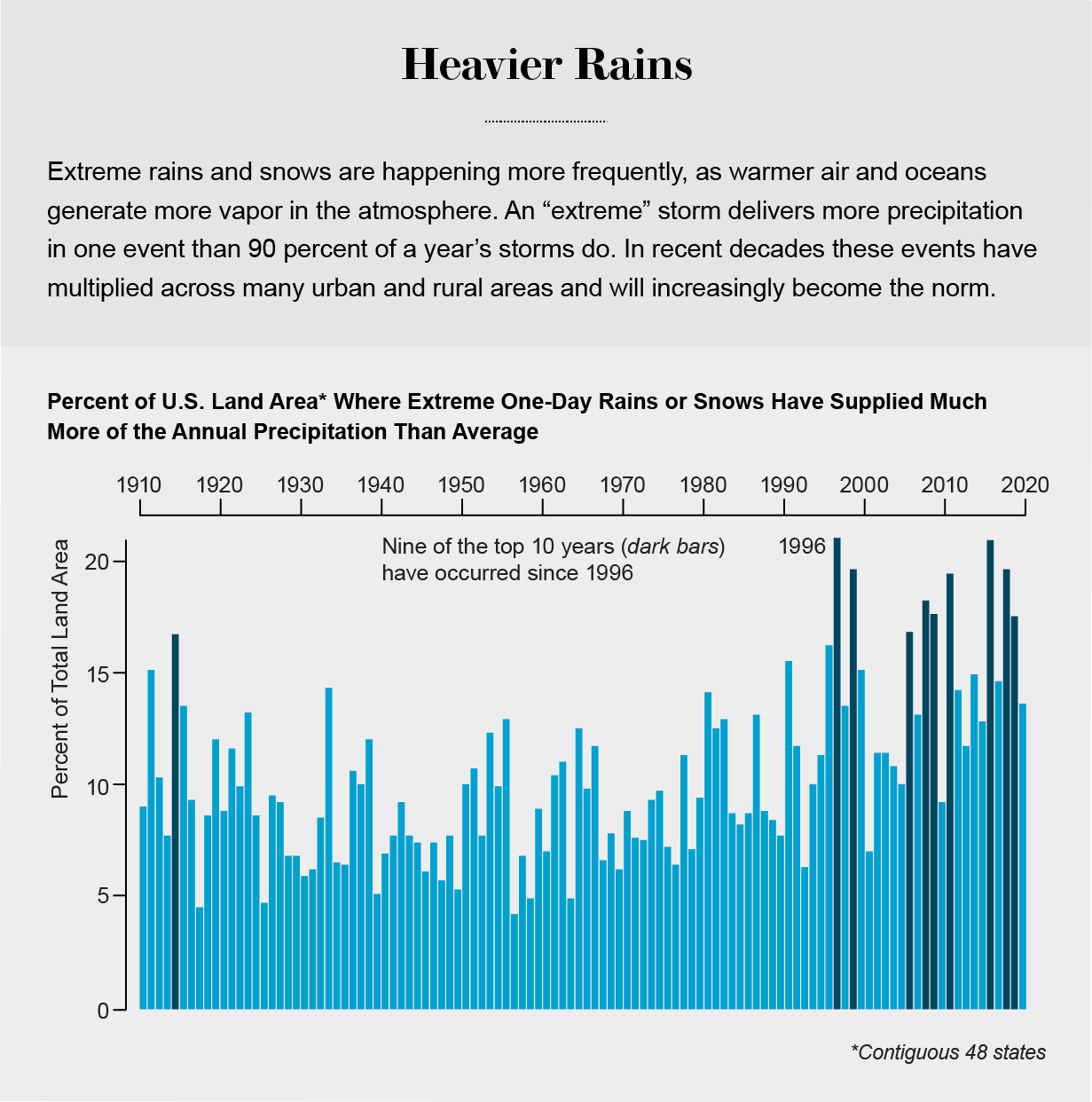
Global Warming Amplifier
If intense storms and sweltering nights are not troubling enough, water vapor is also making global warming worse. Even though carbon dioxide gets most of the attention, water vapor is by far the most important greenhouse gas in the atmosphere. It absorbs much more of the infrared energy radiated upward by Earth’s surface than do other greenhouse gases, thereby trapping more heat. To put this into perspective, a doubling of atmospheric carbon dioxide concentrations by itself would warm the globe approximately one degree C. But feedback loops—vicious cycles—make the temperature rise twice as much. Again, although feedbacks such as disappearing sea ice get a lot of attention, the water-vapor feedback loop—warming causes evaporation, which traps heat, creating even more warming—is the strongest one in the climate system.
Perhaps counterintuitively, the water-vapor feedback is weakest where vapor is most abundant. In humid areas, the infrared energy absorbed by water vapor is already near its physical limit, so adding a little extra moisture has minimal effect. In dry places, however, such as polar regions and deserts, the amount of infrared energy absorbed is well below its potential maximum, so any added vapor will trap more heat and increase temperatures in the lower atmosphere.
The rise in the number and duration of Arctic heat waves is a clear symptom of more frequent and longer-lasting pulses of warm, moist air from lower latitudes—those tendrils that extend northward from the tropics. In January 2021, for example, temperatures soared 36 degrees F (20 degrees C) higher than normal across large areas of the Arctic Ocean. Increasing Arctic heat waves, especially during winter, are slowing the annual freeze-up of sea ice and contributing to the demise of the ice cover.
The heat-trapping effect of additional vapor could perhaps be offset by an increase in cloud formation. Clouds reflect the sun’s rays (leading to a cooling effect) but also trap heat. Over oceans the cooling effect tends to dominate, but the warming influence wins out at high latitudes. Recent studies suggest that on average over the entire globe, the heating effect is greater, establishing yet another vicious cycle involving water vapor.
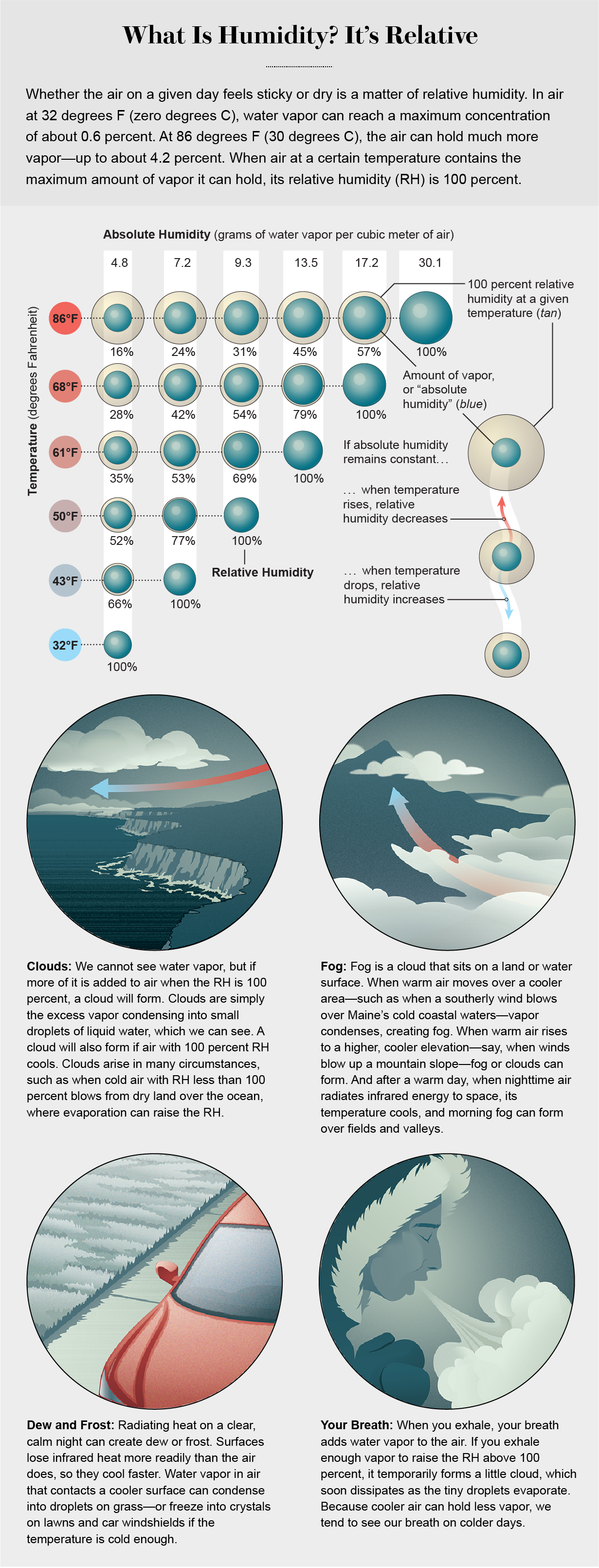
Better Forecasts
As human activities continue to produce more heat-trapping gases, the oceans and atmosphere will continue to warm, and additional water will evaporate, leading to more frequent vapor storms and debilitating steam waves. Hurricanes in the strongest categories will occur more often, as will storms that intensify rapidly. Predicting these quick spin-ups will be a challenge for forecasters. When the storms intensify close to landfall, as Hurricane Ida did, officials will have precious little time to sound the alarm, and people will have mere hours to evacuate.
The main obstacle to predicting these overachieving storms is the lack of temperature measurements below the sea surface. A deep layer of warm water contains a lot more storm fuel than a shallow layer does, but satellites measure only ocean-surface temperatures. Researchers are trying to devise ways to determine how much energy is contained in the upper few hundred feet of ocean water because that is really what storms feed on. They are developing autonomous ocean gliders that roam the upper ocean at different depths while sampling temperature and salinity. They are also working with data from satellites that can detect variations in the height of the sea surface: a deep layer of warm water expands relative to adjacent areas, creating a hump in the ocean surface that can be seen from space.
Satellite data are extremely valuable, but we also need instruments across the ocean that measure temperature, vapor and winds. We will continue to rely on “hurricane hunter” aircraft to fly into storms and drop instruments inside and around them. Researchers feed data from these flights into computer models that can provide details about the atmosphere’s state and the storm’s strength. Better data coverage, faster computers and greater understanding of storm-formation processes are helping to improve predictions.
Vapor arises from myriad sources and affects many atmospheric processes. Scientists do not completely understand some of the interactions, and computer models still struggle to fully predict the influences of water vapor in the changing climate system. Even the seemingly straightforward case of how fast water evaporates from an ocean or lake depends on many factors, such as the difference between the temperature of the water and that of the air just above it, how much vapor is already in the air, and wind speed. Over land the calculation is even more complex, involving additional variables such as how much moisture is in the soil and what types of plants are growing. Predicting what will happen to the vapor once it enters the atmosphere is yet another challenge. Will it condense into clouds, fuel a storm, and fall as rain or snow? Will it condense onto surfaces as dew or frost? Will it travel for hundreds, maybe thousands, of miles from the tropics to higher latitudes? Errors in any of these calculations will affect predictions of future temperature changes and weather patterns.
Increasing water vapor deserves more attention. Unfortunately, we cannot directly control the amount of it in the atmosphere. We can, however, reduce it indirectly by reining in the warming caused mainly by our emissions of carbon dioxide and methane, as well as by the clearing of trees that help to absorb carbon from the air. By reducing the warming rate, we can lessen the surge in vapor. If we succeed, we can slow the future intensification of vapor storms—and the havoc they can wreak.