We trekked through the Bolivian Amazon, drenched in sweat. Draped head to toe in bug repellent gear, we stayed just ahead of the clouds of mosquitoes as we sidestepped roots, vines and giant ants. My local research assistant Dino Nate, my partner Kelly Rosinger and I were following Julio, one of my Tsimane' friends and our guide on this day. Tsimane' are a group of forager-horticulturalists who live in this hot, humid region. Just behind us, Julio's three-year-old son floated happily through the jungle, unfazed by the heat and insects despite his lack of protective clothing, putting my perspiration-soaked efforts to shame.
We stopped in front of what looked like a small tree but turned out to be a large vine. Julio told us Tsimane' use it when they are in the old-growth forest and need water. He began whacking at the vine from all sides with his machete, sending chips of bark flying with each stroke. Within two minutes he had cut off a meter-long section. Water started to pour out of it. He held it over his mouth, drinking from it for a few seconds to quench his thirst, then offered it to me. I put my water bottle under the vine and collected a cup. It tasted pretty good: light, a little chalky, almost carbonated.
As part of my field research, I was asking Julio and other Tsimane' people how they obtain the drinking water they need in different places—in their homes, in the fields, on the river or in the forest. He told me only two types of vines are used for water; the rest don't work or make you sick. But when he pointed to those other vines, I could hardly tell a difference. The vines are a hidden source of water. Julio's observations raise a fundamental question of human adaptation: How did our evolutionary history shape the strategies we use to meet our water needs, particularly in environments without ready access to clean water?
Here in the forest we were in a relatively water-rich environment, but as we moved away from streams, Julio still knew exactly where and how to get water. Humans are not alone in keeping close track of natural water sources—many animals make mental maps of their surroundings to remember where important resources are found, and some even alter their environments for water. But we are unique in taking much more extreme measures.
Throughout history people have drastically engineered their environments to ensure access to water. Take the historic Roman city of Caesarea in modern-day Israel. Back when it was built, more than 2,000 years ago, the region did not have enough naturally occurring freshwater to sustain a city. Because of its geographic importance to their colonial rule, the Romans, through extractive slave labor, built a series of aqueducts to transport water from springs as far as 16 kilometers away. This arrangement provided up to 50,000 people with approximately 145 liters of water per capita a day.
Today cities use vast distribution networks to provide potable water to people, which has led to remarkable improvements in public health. When we have plenty of water, we forget how critical it truly is. But when water is precious, it is all we think about. All it takes is news of a shutoff or contamination event for worries about water insecurity to take hold.
Without enough water, our physical and cognitive functions decline. Without any, we die within a matter of days. In this way, humans are more dependent on water than many other mammals are. Recent research has illuminated the origins of our water needs—and how we adapted to quench that thirst. It turns out that much as food has shaped human evolution, so, too, has water.
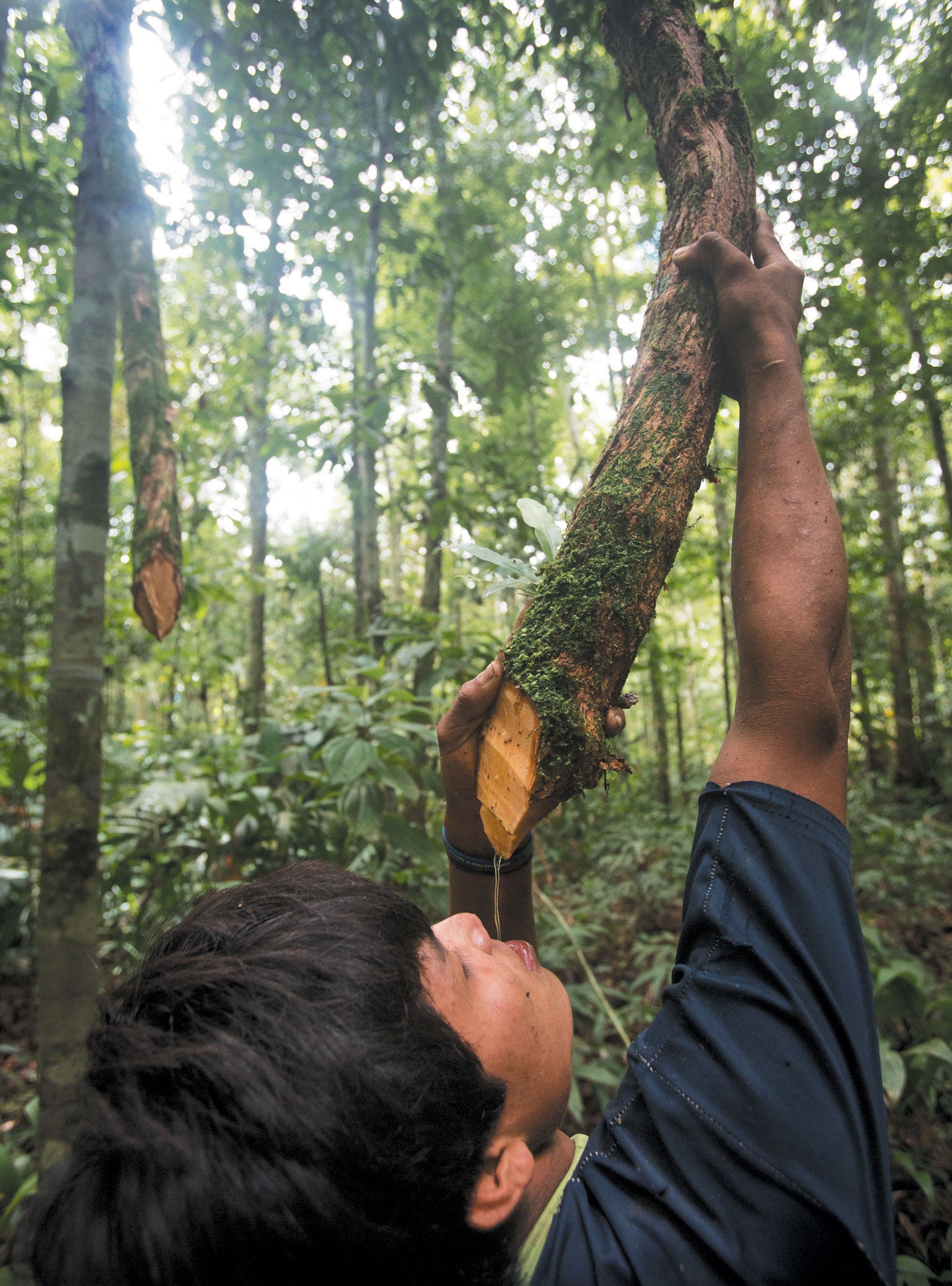
Breaking a Sweat
To understand how water has influenced the course of human evolution, we need to page back to a pivotal chapter of our prehistory. Between around three million and two million years ago, the climate in Africa, where hominins (members of the human family) first evolved, became drier. During this interval, the early hominin genus Australopithecus gave way to our own genus, Homo. In the course of this transition, body proportions changed: whereas australopithecines were short and stocky, Homo had a taller, slimmer build with more surface area. These changes reduced our ancestors' exposure to solar radiation while allowing for greater exposure to wind, which increased their ability to dissipate heat, making them more water-efficient.
Other key adaptations accompanied this shift in body plan. As climate change replaced forests with grasslands, and early hominins became more proficient at traveling on two legs in open environments, they lost their body hair and developed more sweat glands. These adaptations increased our ancestors' ability to unload excess heat and thus maintain a safe body temperature while moving, as work by Nina Jablonski of Pennsylvania State University and Peter Wheeler of Liverpool John Moores University in England has shown.
Sweat glands are a crucial part of our story. Mammals have three types of sweat glands: apocrine, sebaceous and eccrine. The eccrine glands mobilize the water and electrolytes inside cells to produce sweat. Humans have more eccrine sweat glands than any other primate. A recent study by Daniel Aldea of the University of Pennsylvania and his colleagues found that repeated mutations of a gene called Engrailed 1 may have led to this abundance of eccrine sweat glands. In relatively dry environments akin to the ones early hominins evolved in, the evaporation of sweat cools the skin and blood vessels, which, in turn, cools the body's core.
Armed with this powerful cooling system, early humans could afford to be more active than other primates. In fact, some researchers think that persistence hunting—running an animal down until it overheats—may have been an important foraging strategy for our ancestors, one they could not have pursued if they did not have a means to avoid overheating.
This enhanced sweating ability has a downside, however: it elevates our risk of dehydration. Martin Hora of Charles University in Prague and his collaborators recently demonstrated that Homo erectus would have been able to persistence hunt for approximately five hours in the hot savanna before losing 10 percent of its body mass. In humans, 10 percent body mass loss from dehydration is generally the cutoff before serious risk of physiological and cognitive problems or even death occurs. Beyond that point, drinking becomes difficult, and intravenous fluids are needed for rehydration.
Our vulnerability to dehydration means that we are more reliant on external sources of water than our primate cousins and far more than desert-adapted animals such as sheep, camels and goats, which can lose 20 to 40 percent of their body water without risking death. These animals have an extra compartment in the gut called the forestomach that can store water as an internal buffer against dehydration.
In fact, desert-dwelling mammals have a range of adaptations to water scarcity. Some of these traits have to do with the functioning of the kidneys, which maintain the body's water and salt balance. Mammals vary in the size and shape of their kidneys and thus the extent to which they can concentrate urine and thereby conserve body water. The desert pocket mouse, for example, can live without water for months, in part because of the extreme extent to which its kidneys can concentrate urine. Humans can do this to a degree. When we lose copious amounts of water from sweating, a complex network of hormones and neural circuitry directs our kidneys to conserve water by concentrating urine. But our limited ability to do so means we cannot go without freshwater for nearly so long as the pocket mouse.
Neither can we preload our bodies with water. The desert camel can drink and store enough water to draw on for weeks. But if humans drink too much fluid, our urine output quickly increases. Our gut size and the rate at which our stomach empties limit how fast we can rehydrate. Worse, if we drink too much water too fast, we can throw off our electrolyte balance and develop hyponatremia—abnormally low levels of sodium in the blood—which is just as deadly if not more so than dehydration.
Even under favorable conditions, with food and water readily available, people generally do not recover all of their water losses from heavy exercise for at least 24 hours. And so we must be careful to strike a balance in how we lose and replenish the water in our bodies.
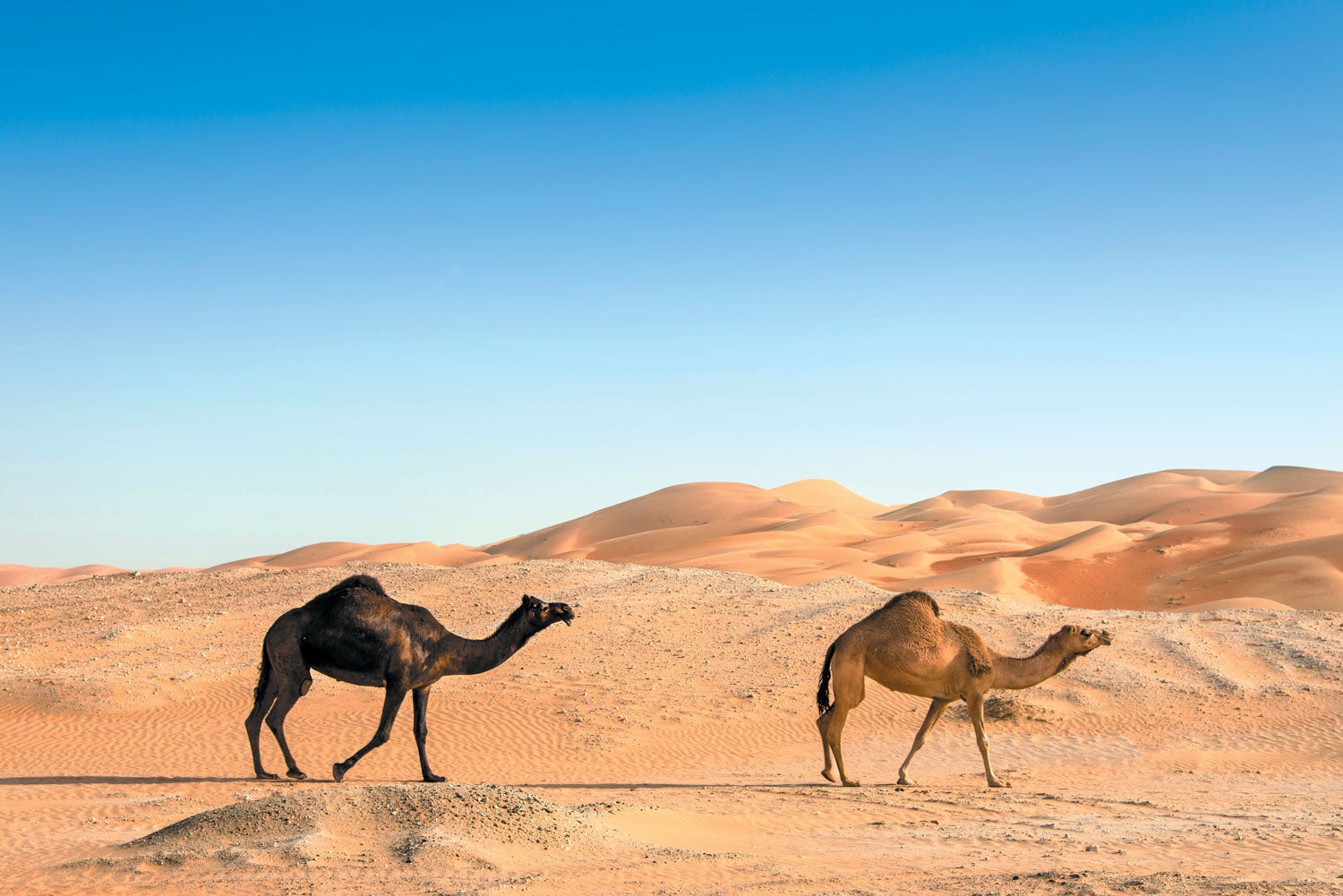
Quenching Our Thirst
There was a reason I was asking Julio about “hidden” sources of water, such as vines, that Tsimane' consumed. One evening after dinner a few weeks into my first bout of fieldwork in Bolivia in 2009, the combination of thirst and hunger led me to devour a large papaya. The juices ran down my chin as I ate the ripe fruit. I didn't think much of it at the moment, but soon after I got into my mosquito net for the night, my error revealed itself.
In the Bolivian Amazon, the humidity reaches up to 100 percent at night. Every evening before going to bed I stripped down to my boxers, then rolled my clothes up tightly and put them into large resealable plastic bags so they wouldn't be soaked the next morning. After about an hour of lying in my mosquito net praying for a gust of wind to cool me off, a dreaded sensation set in: I needed to urinate. Knowing the amount of work it would take to get dressed, relieve myself, and then refold and stow my clothes, I cursed my decision to eat the papaya. And I had to repeat the process again later that night. I started thinking about how much water was in that fruit—the equivalent of three cups, it turns out. No wonder I had to pee.
Our dietary flexibility is perhaps our best defense against dehydration. As I learned the hard way on that sweltering night, the amount of water present in food contributes to total water intake. In the U.S., around 20 percent of the water people ingest comes from food, yet my work among Tsimane' found that foods, including fruits, contribute up to 50 percent of their total water intake. Adults in Japan, who typically drink less water than adults in the U.S., also get around half their water from the foods they eat. Other populations employ different dietary strategies to meet their water needs. Daasanach pastoralists in northern Kenya consume a great deal of milk, which is 87 percent water. They also chew on water-laden roots.
Chimpanzees, our closest living primate relatives, also exhibit dietary and behavioral adaptations to obtaining water. They lick wet rocks and use leaves as sponges to collect water. Primatologist Jill Pruetz of Texas State University has found that in very hot environments, such as the savannas at Fongoli in Senegal, chimps seek shelter in cool caves and forage at night rather than during the day to minimize heat stress and conserve body water. But overall nonhuman primates get most of their water from fruits, leaves and other foods.
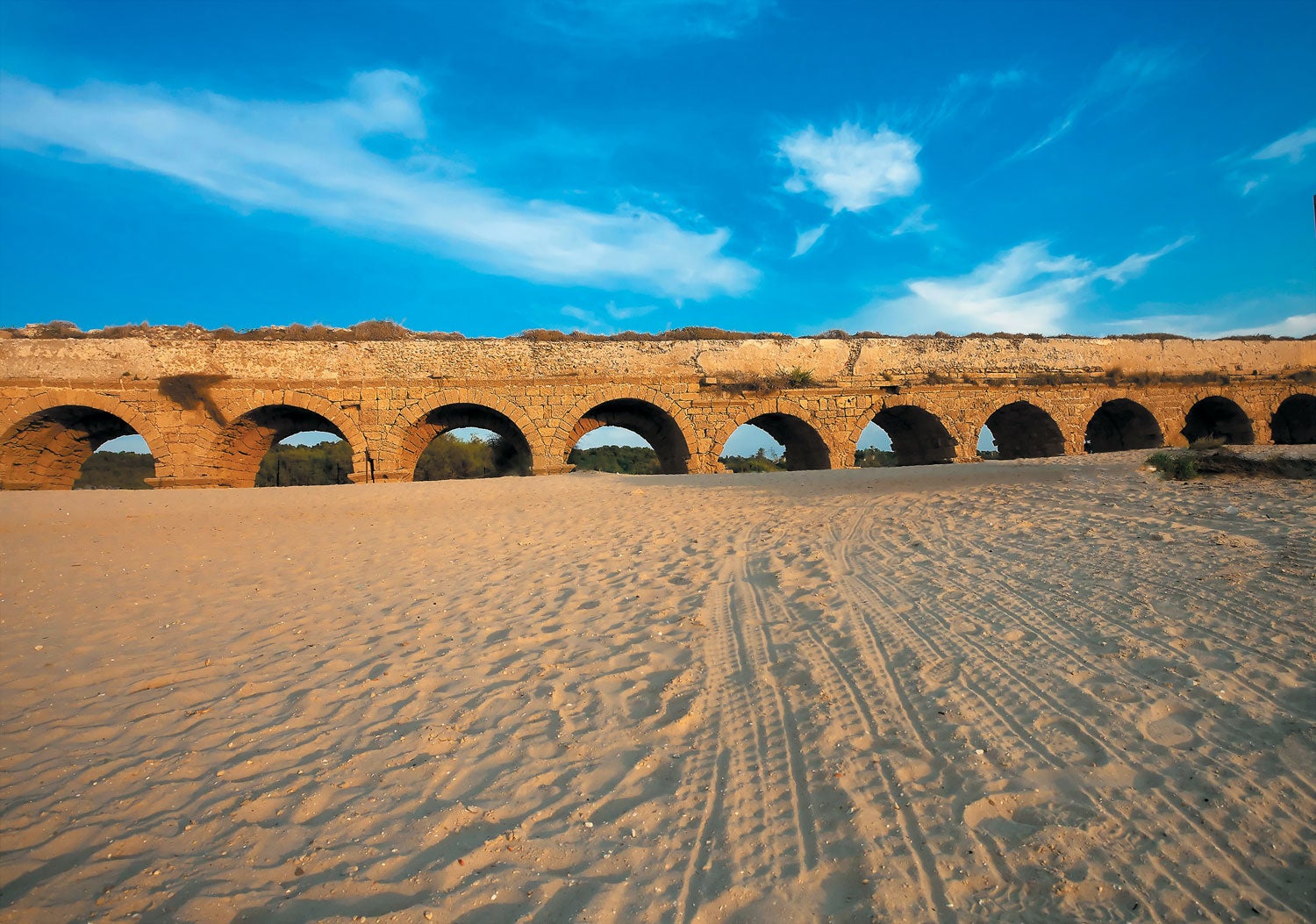
Humans have evolved to use less water than chimps and other apes, despite our greater sweating ability, as new research by Herman Pontzer of Duke University and his colleagues has shown. Yet our greater reliance on plain water as opposed to water from food means that we must work hard to stay hydrated. Exactly how much water is healthy differs between populations and even from person to person, however. Currently there are two different recommendations for water intake, which includes water from food. The first, from the U.S. National Academy of Medicine, recommends 3.7 liters of water a day for men and 2.7 liters for women, while advising pregnant and lactating women to increase their intake by 300 and 700 milliliters, respectively. The second, from the European Food Safety Authority, recommends 2.5 and 2.0 liters a day for men and women, respectively, with the same increases for pregnant and lactating women. Men need more water than women do because their bodies are larger and have more muscle on average.
These are not hard-and-fast recommendations. They were calculated from population averages based on surveys and studies of people in specific regions. They are intended to fulfill the majority of water needs for moderately active, healthy people living in temperate and often climate-controlled environments. Some people may need more or less water depending on factors that include life habits, climate, activity level and age.
In fact, water intake varies widely even in relatively water-secure locations such as the U.S. Most men consume between 1.2 and 6.3 liters on a given day and women between 1.0 and 5.1 liters. Throughout human evolution our ancestors' water intake probably also varied substantially based on activity level, temperature, and exposure to wind and solar radiation, along with body size and water availability.
Yet it is also the case that two people of similar age and physical condition living in the same environment can consume drastically different amounts of water and both be healthy, at least in the short term. Such variation may relate to early life experiences. Humans undergo a sensitive period during fetal development that influences many physiological functions, among them how our bodies balance water. We receive cues about our nutritional environment while in the womb and during nursing. This information may shape the offspring's water needs.
Experimental studies have demonstrated that water restriction among pregnant rats and sheep leads to critical changes in how their offspring detect bodily dehydration. Offspring born to such water-deprived mothers will be more dehydrated (that is, their urine and blood will be more concentrated) than offspring born to nondeprived mothers before they become thirsty and seek out water. These findings indicate that the dehydration-sensitivity set point is established in the womb.
Thus, the hydration cues received during development may determine when people perceive thirst, as well as how much water they drink later in life. In a sense, these early experiences prepare offspring for the amount of water present in their environment. If a pregnant woman is dealing with a water-scarce environment and is chronically dehydrated, it may lead to her child consistently drinking less water later in life—a trait that is adaptive in places where water is hard to come by. Much more work is needed to test this theory, however.
Keeping It Clean
Although early life experiences may determine how much water we drink without our being aware of it, locating safe sources of water is something we actively learn to do. In contrast to my accidental discovery of the hydrating effects of the papaya, Tsimane' deliberately seek out water-rich foods. In an environment without clean water, eating instead of drinking more water may protect against exposures to pathogens. Indeed, my study found that those Tsimane' who consumed more of their water from foods and fruit, such as papayas, were less likely to experience diarrhea.
Many societies have developed dietary traditions that incorporate low-alcohol, fermented beverages, which can be essential sources of hydration because fermentation kills bacteria. (Beverages containing higher percentages of alcohol, on the other hand, increase urine production and thereby deplete the body's water stores.) Like other Amazonian populations, Tsimane' drink a fermented beverage called chicha that is made from yuca or cassava. For Tsimane' men, consuming fermented chicha was associated with lower odds of becoming dehydrated.
Getting enough water is one of humanity's oldest and most pressing challenges. Perhaps it is not surprising, then, that we map the locations of water sources in our minds, whether it is a highway rest stop, desert spring or jungle plant. As I watched Julio cut the vine down, his son was also watching, learning where this critical water source was. I glimpsed how this process plays out across generations. In so doing, I realized that being covered in sweat and finding ways to replace that lost water is a big part of what makes us human.