She was born in the usual way. A disorganized blob of clouds emerged over the Atlantic Ocean off Africa’s bulging western coast, just north of the equator. Atmospheric pressure there was low, typical for late summer. Natural variability in the earth’s climate spawns tropical disturbances every year in this area—sometimes more, sometimes fewer, and sometimes they become hurricanes. Weather forecast models unanimously predicted that the clouds would coalesce into a storm that curved harmlessly northwest into the mid-Atlantic, far from land.
On September 1, 2018, tropical storm Florence began to do just that but then turned stubbornly westward while becoming better organized, seemingly aimed at the Caribbean. Another worrisome disturbance was already lurking near Puerto Rico, which was still reeling from Hurricane Maria’s devastation a year before. Three major cyclones were also spinning in the tropical Pacific, fueled by ocean temperatures that were off the charts. The mob of storms drew energy from record-warm ocean temperatures, which have risen steadily since the 1970s in lockstep with increasing atmospheric temperatures, driven by heat-trapping greenhouse gases—global effects of climate change. Storms feed of ocean heat and water vapor in the atmosphere, which is climbing as well.
Conflicting factors kept Florence weak, however. Although that seemed fortunate, it provoked anxiety among forecasters. Weaker storms are more readily steered by lower-altitude winds, and those winds were blowing east to west, straight at the U.S. East Coast. They were skirting the southern rim of a big, circular, unusually strong high-pressure center parked in the middle of the North Atlantic. High-pressure centers arise naturally, but evidence shows that some are getting stuck in place more often, a regional symptom of a warmer climate. A “blocking high” like this one had steered Hurricane Sandy on its bizarre path from the Atlantic into New Jersey in 2012.
On September 4 something unexpected happened: weak Florence moved over a spot in the west-central Atlantic that was abnormally warm. Fueled by that warmth, she rapidly intensified to a Category 4 hurricane and at a nearly record-breaking northerly latitude. Random hot pools of ocean water are another regional effect of our changing climate.
As Florence churned, the forecast models began to coalesce: she seemed likely to hit the coastal Carolinas and stall, where she could unleash deep flooding as Hurricane Harvey had done a year earlier over Houston. Sure enough, Florence struck the coastal Carolinas on September 14 and sat for four days. The low-altitude steering winds were now too weak to move the system. Florence dropped more than 30 inches of rain in places and reportedly killed more than 50 people and millions of animals (mostly chickens) while racking up an estimated $20 billion or so in losses. Floodwaters passing through factory farms, mines and sewage-treatment plants polluted rivers and estuaries for weeks.
Florence’s wrath will not soon be forgotten, and neither will the blatant demonstration of climate change in action. The hurricane’s unusual severity can be attributed to specific effects of climate change: greater heat in the air and ocean, extra water vapor, the tenacious blocking high and weak steering winds. These factors are in play around the world, favoring rapid storm intensification, heavier precipitation, greater flooding and stronger storm winds.
Florence was just one of many examples in 2018 of the various ways climate change is afecting extreme weather. Multiple “bomb cyclones” battered the northeastern U.S. An outbreak of Arctic air called the “beast from the east” froze Europe. Severe heat waves crippled Japan, Scandinavia and Greece. Floods ruined parts of Venice, Paris and Maryland.
Such misfortunes have happened since humans walked the earth, of course. But every year it becomes clearer that today’s epidemic of bizarre weather cannot be explained by natural variability. Although in the past scientists were careful to not directly link climate change to specific weather events, we are now indeed saying that because of climate change, major floods are occurring more often. Killer heat waves are hotter and lasting longer. Cold spells are sticking around longer in some places, too.
How much of our daily wild weather, which in 2018 caused more than $160 billion in losses worldwide, can be blamed on changing climate? The answer depends on untangling the roles of three broad factors. First is the global efect of more heat in the ocean and more heat and vapor in the atmosphere. The water vapor story deserves more attention than it gets: vapor is another greenhouse gas that traps heat, it releases even more heat when it condenses into clouds, and it feeds precipitation from storms.
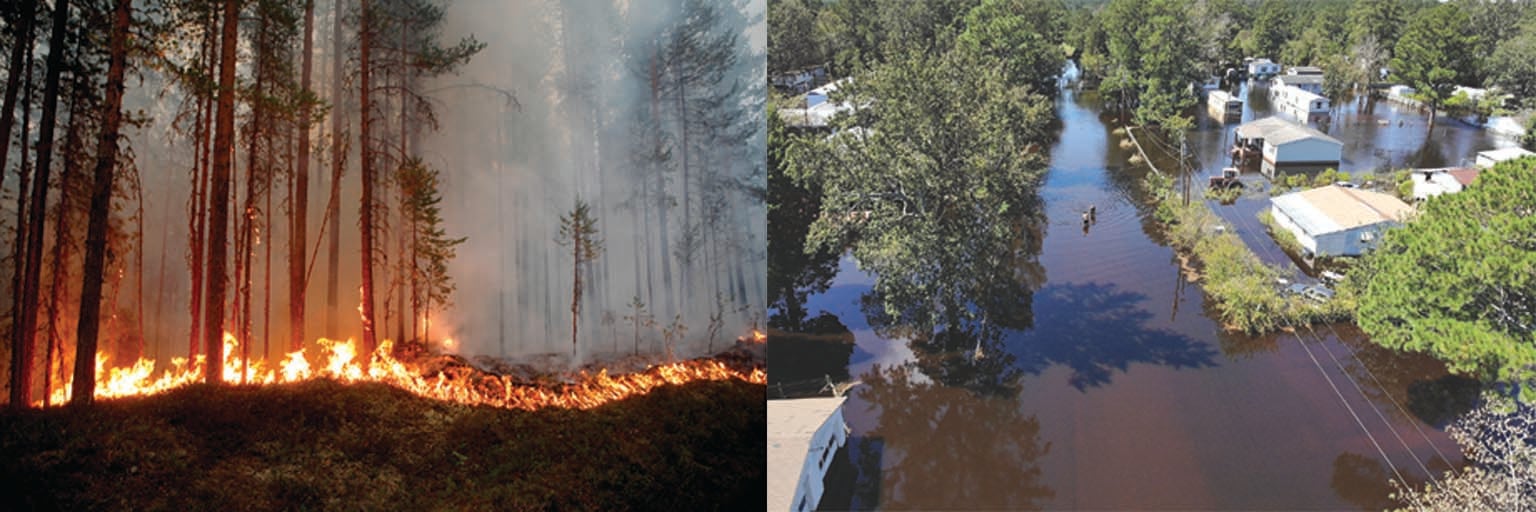
The second factor is regional effects, such as stubborn, blocking high-pressure centers, expanses of melting sea ice, a growing “cold blob” of ocean water south of Greenland, a slowing Gulf Stream and an increasingly disrupted polar vortex.
The third involves the complicated ways that natural variations, such as El Niño and La Niña, interact with the regional factors. Research into this interplay is cutting-edge, controversial—and bearing fruit. We now understand better how and why climate change is escalating extreme weather, revealing how we will need to prepare for increasingly frequent and intense dangers.
A Nasty Nor’Easter
The earth’s atmosphere is a cloak of roiling gases. Air constantly heats and cools, with the sun pouring in energy during the day and warm surfaces radiating it back to space at night. Uneven heating creates local winds that blow every which way. Water is continuously evaporating from land and sea, condensing in the air and falling down as rain or snow.
Yet within this chaos are remarkably predictable patterns governed by latitude, the earth’s spin, mountain ranges, ocean circulations and other influences. In the Atlantic, hurricanes like Florence form in the eastern tropics and move westward. In the Pacific, tropical storms move westward, too. A polar jet stream blows from west to east around the Northern Hemisphere at a latitude near the U.S.-Canada border; another polar jet in the Southern Hemisphere crosses the lower reaches of South America and Africa. More cyclical are large wind systems linked to ocean-temperature fluctuations, such as El Niño and La Niña, which wax and wane every three to eight years and affect winds and precipitation worldwide. Data from cores of mud extracted from various seabeds show these patterns have held for hundreds of thousands of years.
Chaos and consistency also prevail in the oceans, over longer cycles, amid constant heating, cooling and flowing waters. The Pacific Decadal Oscillation is a seesawing of warm and cold temperatures between the eastern and western North Pacific Ocean, each phase persisting for about a decade. The Atlantic Meridional Overturning Circulation is a slow current of warm, salty surface water that heads north in the western Atlantic, then loses heat, dives deep and flows back south to Antarctica. A round-trip through this circuit takes about 1,000 years.
Interactions between the atmosphere and oceans introduce natural variability into climate. Some years are hotter or colder in certain regions; some years are wetter or drier. But those same mud-core data from past millennia show that the variability has limits: the climate rarely exceeds certain bounds. Until now.
I experienced one of those exceptions firsthand in the late winter of 2018. March is supposed to roar in like a lion, but this was ridiculous. Mother Nature was delivering a parade of potent nor’easters—storms that churn just off the Atlantic Coast, lashing the Eastern Seaboard with winds from the northeast. The three climate change factors—higher heat and vapor globally, regional effects, and the interplay between natural variability and those effects—are goosing these storms.
I was anxiously monitoring the latest forecast models. They showed a seemingly innocuous wiggle in the jet stream over the North Pacific, and they agreed that it would cross to the Atlantic Seaboard and spawn a whopper of a storm aimed at our coastal town in southeastern Massachusetts. The models were bullish about a blizzard developing quickly—technically a bomb cyclone—and dumping its snow right on my neighborhood. It would be the third major nor’easter of the season, which is unusual.
All the ingredients were in place. Cold air was entrenched over Eastern states (a naturally occurring factor). Extra heat in the ocean (a global factor) provided ample energy and moisture for a mounting storm. Water temperatures in the Atlantic off New England were far above normal (a regional factor).
The interplay between natural and regional factors was another important ingredient. In late 2013 the Pacific Decadal Oscillation flipped from its so-called negative phase to a positive phase, in keeping with its natural cycle. It raised sea temperatures above average along the western coast of North America. Extra heat pumped into the atmosphere from this warm water favors the formation of a northward bulge (called a ridge) in the jet stream over western North America that can extend into Alaska.
That is where interaction with a regional effect comes into play. Air temperatures in the Arctic have risen two to three times faster than the rest of the planet, especially in winter. The extraordinary loss of 75 percent of the Arctic’s sea-ice volume in only 40 years is responsible for most of this warming. The Pacific ridge can tap into this extra warmth, causing it to intensify and stick around for a long time. This so-called Ridiculously Resilient Ridge is largely responsible for the extended drought and heat waves that set the stage for recent severe wildfires along the U.S. West Coast.
A strong ridge is usually accompanied by a large southward dip (called a trough) to the east of it, which in this case was over eastern North America. A deep trough allows cold Arctic air to plunge far southward, creating a stark temperature contrast with the warm Atlantic waters along the East Coast. The atmosphere despises temperature contrasts. It generates storms to mix air masses in an attempt to even out the differences; bomb cyclones are an intense example of this process. This ridge-and-trough pattern tends to spawn nor’easters, and it has become increasingly prevalent in recent winters.
Sure enough, National Weather Service data painted a scenario of “bombogenesis”—when a storm’s atmospheric pressure drops more than 24 millibars in 24 hours, causing it to “explode” in size and power. My neighborhood was in the crosshairs. As dusk arrived on March 2, so did the nor’easter’s howling wind, driving rain and snow, power outages, and major erosion from high waves and storm surges. Afraid that one of the tall white pines in our yard might fall on the house, my cat and I (my husband was away) opted to sleep on the living-room couch rather than in my upstairs bedroom. The wind roared so loudly overnight that I did not hear the crash of any of the 20 big trees that dropped around us, somehow missing our roof.
The storm took its sweet time leaving, as a blocking high-pressure center near Greenland thwarted its movement, ravaging half a dozen states with hurricane-force winds. The nor’easter killed at least nine individuals, knocked out power for more than two million people (five days in our town) and flooded coastal communities.
Wicked winter weather
The parade of destructive nor’easters was not the only winter weather in 2018 juiced by climate change. Parisians and Venetians suffered the worst flooding in half a century as a result of prolonged rainfall, while deadly windstorms struck Germany and northern France. Several feet of snow buried Davos, Switzerland, just as the well-heeled and high-heeled tried to arrive for the annual meeting of the World Economic Forum.
In North America, the big story was “weather whiplash”—sudden and dramatic shifts between long-lasting extremes. Although research is still sparse, evidence is accumulating that these exaggerated swings are occurring more frequently and that our three climate influences are in play.
Case in point: during three weeks of January 2019, such bitter cold gripped the eastern U.S. that iguanas in southern Florida dropped from trees in near-frozen comas while residents in Western states basked in above-normal temperatures. Then in early February, weather whiplash struck. An abrupt reversal in the jet-stream pattern brought record-breaking warmth to hundreds of Eastern cities. Temperatures jumped more than 40 degrees Fahrenheit in 24 hours, bringing the iguanas back to life. At the same time, a deep chill settled over the Western states. In the atmospheric battleground between the eastern and western air masses, potent storms in the Mississippi Valley caused the worst flooding in decades. The frequency of heavy precipitation in that region has increased by about 40 percent since the 1950s.
Global, regional and interplay factors had struck again. Overall global warming and moistening certainly gave these extremes a boost. And the same regionally loopy jet stream that would contribute to the parade of bomb cyclones had set the stage. Winter whiplash slapped the U.S. and Canada in February 2019, too; in some areas, temperatures ricocheted by 50 or 60 degrees F and wind chills by more than 100 degrees F in only a few days.
Scorching, soaking summer
Summer 2018 also brought a smorgasbord of rough weather to the Northern Hemisphere, much of it exacerbated by climate change. While Japan, Texas and even Scandinavia baked for weeks, the U.S. Eastern Seaboard sloshed through its wettest season on record. Tenacious droughts plagued the western U.S., parts of Europe and the Middle East, contributing to a horrific spate of wildfires that cost $20 billion in California alone. Extreme summer conditions ruined crops, boosted toxic algae blooms, shut down nuclear-reactor cooling systems and triggered blackouts across four continents.
Some impacts were clearly related to the global factor. Higher average temperatures cause hotter heat waves. Extra water vapor feeds summer downpours and helps to raise nighttime temperatures by trapping additional heat near the surface. The exceptional heat plus humidity, especially at night, can be a deadly combination, making it difficult for the human body to cool itself through evaporation of sweat. Worldwide, thousands of people without air-conditioning died.
Less straightforward were climate influences on the summer jet stream—literally a “hot” research topic. What is already clear, though, is that both global and regional factors are involved in favoring an unusually wavy jet stream such as the one that engulfed Scandinavia in heat waves, drought and fire. Temperatures there from May through July in 2018 broke records going back 260 years.
What role did regional changes play? During spring and summer, warming occurs across a band of land south of Canada and Russia’s Arctic coasts. Spring snow cover has been melting there ever earlier. The loss of this highly reflective surface exposes the underlying soil to strong spring sunshine sooner, drying it out earlier. Dry soil warms much faster than damp soil, so temperatures climb. The warm-up gives summer a head start, shifting the jet stream northward sooner than usual, allowing warm air to penetrate high latitudes.
The band of abnormally warm land can help split the jet stream into two branches, a common occurrence during winter but less so in summer. Weather systems between the two often get trapped for long periods because there is little wind there to move them along. During the summer of 2018 the jet stream over Eurasia and North America was split much of the time, creating persistently warm, dry conditions in some areas, and prolonged rainy periods in others, that broke records on both continents.
Fasten your seat belt
The wild weather of 2018 was a trailer for the main feature, coming to audiences everywhere as greenhouse gases continue to accumulate. Some of the consequences of global effects—warmer oceans, warmer air and increased atmospheric moisture—are obvious and direct. Intense research is focused on untangling the jumble of regional effects and their interplay with natural variability. Let’s look at four examples.
Evidence suggests that the earth’s tropical zone around the equator has been widening toward the poles. That pushes storm tracks poleward and makes some temperate regions hotter and drier. The clearest symptoms can be seen in the dry zones that define the northern and southern edges of the tropics, such as southern California, the Mediterranean and Australia, where more severe droughts and heat waves have captured headlines. Scientists are working to understand the likely contributors—warming, atmospheric dust and soot particles that alter air temperatures and cloud formation.
Another regional factor under scrutiny is the apparent slowing of the Gulf Stream, a major ocean current flowing from the Gulf of Mexico up the Eastern Seaboard, then across the North Atlantic toward the U.K. It is the surface branch of the larger Atlantic Meridional Overturning Circulation. A slowdown would upset weather patterns and fisheries on both sides of the ocean. Measurements of the subsurface ocean are sparse, but oddities in well-monitored surface temperatures, such as abnormal warmth along the East Coast and the blob of cool waters south of Greenland, provide important clues that this large-scale circulation system may indeed be slowing. A shift in ocean-temperature patterns will alter the strength and path of storms. A hyperactive North Atlantic storm track in recent years may be a response to a slowing Gulf Stream, along with feverish waters that most likely fomented the bomb cyclones of the winter of 2018.
Blocking high-pressure centers are another regional feature to watch. Observations suggest they are occurring more often in some places, such as over Greenland and western Russia, but atmospheric models struggle to predict the development and demise of these swirling eddies in the sky. Blocks can form for various reasons, some related to natural variability and some to climate change. The remnants of tropical storms, for example, can naturally inject surges of energy into the jet stream, causing it to buckle and spin off orphan eddies. As oceans warm, however, tropical storms may survive farther northward and later into the autumn season, increasing the likelihood of collisions with the jet stream that can create a block, which can then push hurricanes and other weather systems in unusual directions.
During October 2018, for example, Hurricane Leslie dawdled in the Atlantic for more than two weeks, finally going where no known hurricane has gone before: just west of Portugal. Strong winds and flooding rains pummeled the Iberian Peninsula. At the time, a strong block sat over northeastern Europe, creating the wavy jet-stream pattern that captured Leslie and carried it on a long journey across the Atlantic.
A final regional factor is the stratospheric polar vortex, which was in the news frequently in the winter of 2018—and again in the winter of 2019. It has been behaving oddly. This ring of strong winds circles a pool of frigid air over the North Pole only in winter, at an altitude of around 30 miles. Every few years the right conditions can deform the ring or even split it into two or more smaller rings, which tend to migrate southward, bringing severe cold spells with them. Simultaneously, warm air from the south invades the Arctic, creating topsy-turvy temperatures. During the split polar vortex in late January 2019, it was warmer near the North Pole than in Chicago. These so-called sudden stratospheric warming events can occur naturally, but lately they are happening more often. Several new studies have found that the dramatic sea-ice loss in the Arctic Ocean north of western Russia may help trigger these vortex disruptions. Those of us living in the midlatitudes may be attacked more frequently by the polar vortex as global warming intensifies.
Although certain aspects of the uncontrolled experiment we are forcing on the earth’s climate remain elusive, science is rapidly revealing that climate change can be blamed for amplifying extreme weather and its consequences. Understanding the links will help us see the future more clearly and prepare for the impacts on agriculture, international security, marine life, forests, freshwater resources, infrastructure and human health. The effects are already apparent and will only worsen.
Yet there is reason for hope. The rash of bad weather has shined a bright light on the well-funded campaign to spread disinformation and generate doubt about climate change among the public. Despite what the doubters say, natural variability simply cannot explain the extremes we already see and feel. Recent surveys show most people finally accept that climate change is real and caused by us. Insurers, military leaders, property developers and municipal administrators are responding to the tangible risks to life and property. Perhaps we are finally ready to confront the rough ride ahead.