Stand atop the steep white cliffs that surround the giant rivers of Siberia, and your feet will mark a pivotal point in the history of life on Earth: the 541-million-year-old geologic boundary between the Precambrian and Cambrian periods. The rocks below this dividing line contain scant fossil remains—ghostly impressions of soft-bodied organisms and a smattering of shelly forms. But break open any of the rocks just above the boundary, and they will be teeming with shells. A little higher up still, familiar fossil creatures such as trilobites appear. These changes document the so-called Cambrian explosion, one of the most significant, but still poorly understood, events in all of evolution.
For decades scientists thought that complex animals—multicellular organisms with differentiated tissue types—originated in the Cambrian explosion. To be sure, a riot of novel forms burst into existence during this time, including the ancestors of many of today’s major animal groups. But recent discoveries from Siberia, Namibia and elsewhere show that complex animals actually got their start millions of years before the Cambrian explosion, during the last chapter of the Precambrian, known as the Ediacaran. Among these finds are the oldest known creatures with external and internal skeletons composed of mineralized tissue, a pivotal evolutionary innovation seen in many modern-day animals.
The presence of these armored creatures so far back in time—550 million years ago—indicates that the ecological and environmental pressures thought to have driven the Cambrian explosion were in fact at work long before then. Figuring out how these factors shaped the evolution of the earliest complex animals in the Ediacaran is key to understanding the astonishing burst of diversification that followed in the Cambrian.
The Cambrian fossil record has been the subject of intense study for more than 150 years. Thus, the broad global patterns of what Cambrian fossils appeared when—and where—are relatively well established: similar fossils turned up on many continents at around the same time, and they followed the same succession of evolutionary changes more or less synchronously. But only now, with the discoveries of the older Ediacaran fossils, are we starting to see the roots of the Cambrian explosion.
Gratifyingly, we are also beginning to puzzle out why it happened when it did, thanks in part to the development of new geochemical techniques that have revolutionized our understanding of the changing chemistry of the oceans in the Ediacaran-Cambrian world. Insights from the emerging fossil and geochemical records have just recently been integrated to show how the planet’s biosphere, geosphere, hydrosphere and atmosphere—together known as the Earth system—may have operated during this interval. But already we can paint a striking picture of how the seafloor became successively populated by ever more complex creatures tens of millions of years before the Cambrian explosion, setting the stage for the rise of animal life as we know it.
The first animals
The oldest possible evidence of ancient animals comes not from recognizable fossils but from the remains of organic compounds known as biomarkers. Researchers have found one such biomarker, a particular form of sterane, in very well preserved rocks from a sedimentary sequence known as the Huqf Supergroup in Oman, which is at least 650 million years old. Some experts have argued that these steranes are unique to a particular group of sponges and that the presence of the molecules in the Huqf rocks therefore documents the existence of these animals at that very early time. Not all scientists accept the assertion that these steranes are specific to those sponges, however. Indeed, a study published in April suggests that they are diagnostic of a group of single-celled forms of amoebas.
The oldest candidate animal fossils, which hail from a sequence of rocks in southern China called the Lantian Formation and are possibly as old as 635 million years, are similarly contested. Some investigators think these tiny, soft-bodied forms are related to corals or jellyfish because they exhibit tentaclelike structures, but the preservation of these fossils is not sufficiently clear to allow unequivocal interpretation, leaving many researchers unconvinced that they represent animals of any kind.
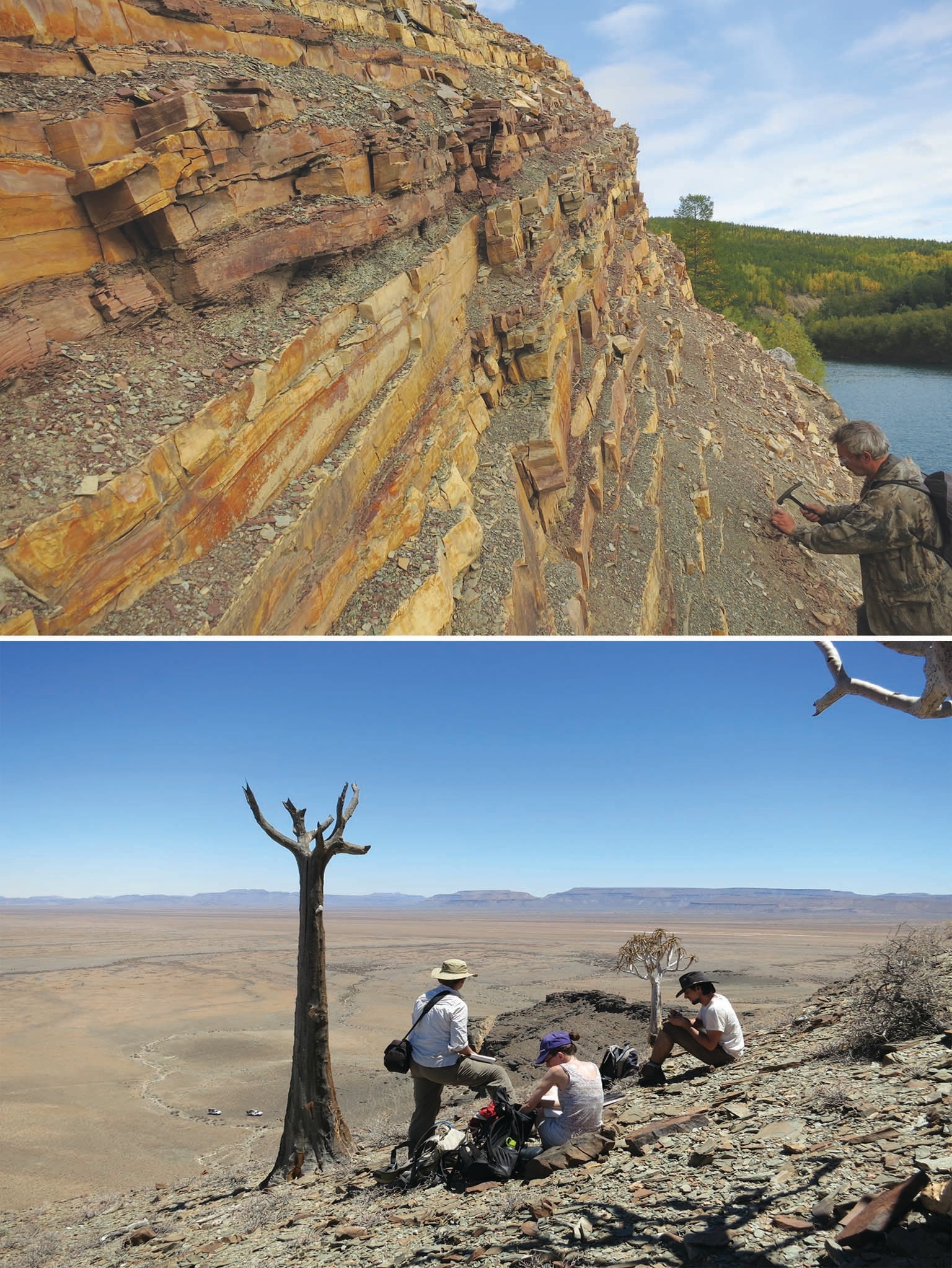
The oldest animal remains that almost everyone can agree on are fossils from Newfoundland that date to about 571 million years ago, shortly after the last regional "Snowball Earth" glaciation that encased much of the planet in thick ice. These earliest known representatives of the Ediacaran biota were dominated by soft-bodied creatures up to a meter in height or width. Some took the form of large, featherlike fronds with vertical stalks that rooted them to the seafloor; others sprawled across the ocean bottom, their flat bodies exhibiting a fractal architecture, with branching units that showed the same patterns at all scales. All these body plans maximize surface area, suggesting that these animals absorbed nutrients directly from the surrounding water.
This modest variety of fauna prevailed for more than 10 million years. But then the pace of animal evolution began to accelerate. The fossil record indicates that after around 560 million years ago, the Ediacaran biota diversified to include mobile forms that inhabited shallow seas. Some of the fossils preserve scratch marks that suggest the animals were eating algal mats by grazing. Others may have dragged themselves across the algae, absorbing nutrients from the underside of their bodies. The first simple burrows also appear at around this time, evidence that animals had started to move and disturb the sediment of the seafloor.
Fast-forward to around 550 million years ago, and the oldest fossils preserving external and internal skeletons suddenly appear in limestone rocks (which consist mainly of calcium carbonate). These fossils are already diverse in size and form, and they show up in such far-flung locales as Siberia, Brazil and Namibia. The presence of skeletons in so many unrelated animal groups around the world at this point in time is testament to a major driving evolutionary force operating on a global scale. We do not know for sure what this force was. But we have an idea. Making a skeleton is energetically expensive, so for an animal to undertake such an endeavor the benefit must outweigh the cost. Animals may produce a skeleton for many reasons, but by far the most common is the need for protection from predators. Although there is no fossil evidence of predators from this time period, it stands to reason that the appearance of skeletons might reflect the first widespread occurrence of animals that ate other animals.
Stronger together
Recent analyses of these ancient skeletons have yielded tantalizing clues to what their owners looked like—and how they lived. Known from fossils of its delicate tubular skeleton that grew up to about 70 millimeters long and resembled a stack of ice cream cones, an organism called Cloudina has figured importantly in our reconstructions of Ediacaran ecosystems. Cloudina was first discovered in Namibia in 1972 and was long assumed to have grown attached to the seafloor. But in the past few years researchers have identified many new specimens of Cloudina from sites around the world that have changed that view. My team’s work on specimens from Namibia has shown that Cloudina had a variety of growth styles. It could attach to mats made of microbes that bound the soft sediment of the seafloor, or it could anchor itself to layered mounds of cyanobacteria. Most important of all, Cloudina individuals could actually cement themselves to one another to form a reef. This finding has established Cloudina as one of the oldest reef-building animals, pushing back the record of this way of life by some 20 million years.
Whether Cloudina was related to modern reef builders such as corals remains uncertain. But we do know that like reef-building corals, it lived in proximity to a number of other animals. Hints of this intimate association have come from other skeletal fossils found in rocks of the same age as those that contain Cloudina fossils. A creature called Namacalathus, known from fossil localities around the world, appears to have been one of Cloudina’s consorts. Its skeleton was up to 50 millimeters long, composed of a delicate, thin-walled stalk and a cup with a central opening at the top and several openings around the sides. The animal’s soft tissue was probably mainly inside the cup, although it is never preserved. Fossils of Namacalathus indicate that it grew rooted to microbial mats, often near Cloudina.
Namapoikia, a creature known only from fossil localities in Namibia, also fraternized with Cloudina. This animal is remarkable for its large size—up to one meter in diameter—and robust skeleton. On the basis of its growth form, we think Namapoikia was a sponge and so would have had an internal skeleton, in contrast to the external skeletons Cloudina and Namacalathus probably had. Intriguingly, Namapoikia grew within the hidden places of reefs, encrusting the vertical walls of open cracks and fissures. In modern reefs the communities of animals and plants that live on open surfaces differ from those that occupy these more hidden areas such as caves, crevices or underhangs. Our Ediacaran fossil discoveries indicate that this distinction is as old as animal reefs themselves.
These observations are significant because reef building represents an important ecological innovation. By growing closely together and even cementing to one another, individuals can become mechanically stronger, rise above the seafloor away from competitors, enhance feeding efficiency and gain protection from predators. Like the earliest skeletons, then, the appearance of reefs in the Ediacaran fossil record may signal rising, complex ecological pressures. The Cambrian explosion, and indeed an arms race between predator and prey, had already begun.
The ediacaran world
By the mid-2010s it was becoming clear that the Cambrian did not mark the sudden, dramatic departure from the Ediacaran that experts long envisioned. Not only had researchers begun to amass evidence that animals started evolving skeletons and building reefs earlier than traditionally thought, but we had also developed ecosystem models showing that Ediacaran animal communities shared many ecological traits with Cambrian ones. The "explosion," we were learning, had a far longer fuse than was previously recognized.
Then, a few years ago, some key discoveries in Siberia and China blurred the Ediacaran and Cambrian worlds even further. A group of researchers from China and Germany found that Cloudina persisted into the Cambrian. And my group, together with colleagues from Russia and China, found fossils long thought to be unique to the Cambrian in Ediacaran rocks. These findings underscored to us that to solve the mystery of the Cambrian explosion we had to figure out the dynamics of the Ediacaran world in which these animals originated.
The possible role of shifting oxygen availability is one dynamic that researchers have been particularly keen to pin down. Animals need oxygen, so a central debate over the past few years has been to understand whether at some point in the time spanning the Ediacaran and Cambrian oxygen levels rose beyond a certain critical threshold, allowing animals to flourish. The question is more complicated than it might seem because animals do not all have the same oxygen requirements. Simple, immobile creatures, such as sponges, may need less oxygen than mobile animals, and they certainly require far less of the stuff than active, fast-swimming predators do. We have borne this variation in mind in the course of our investigations.
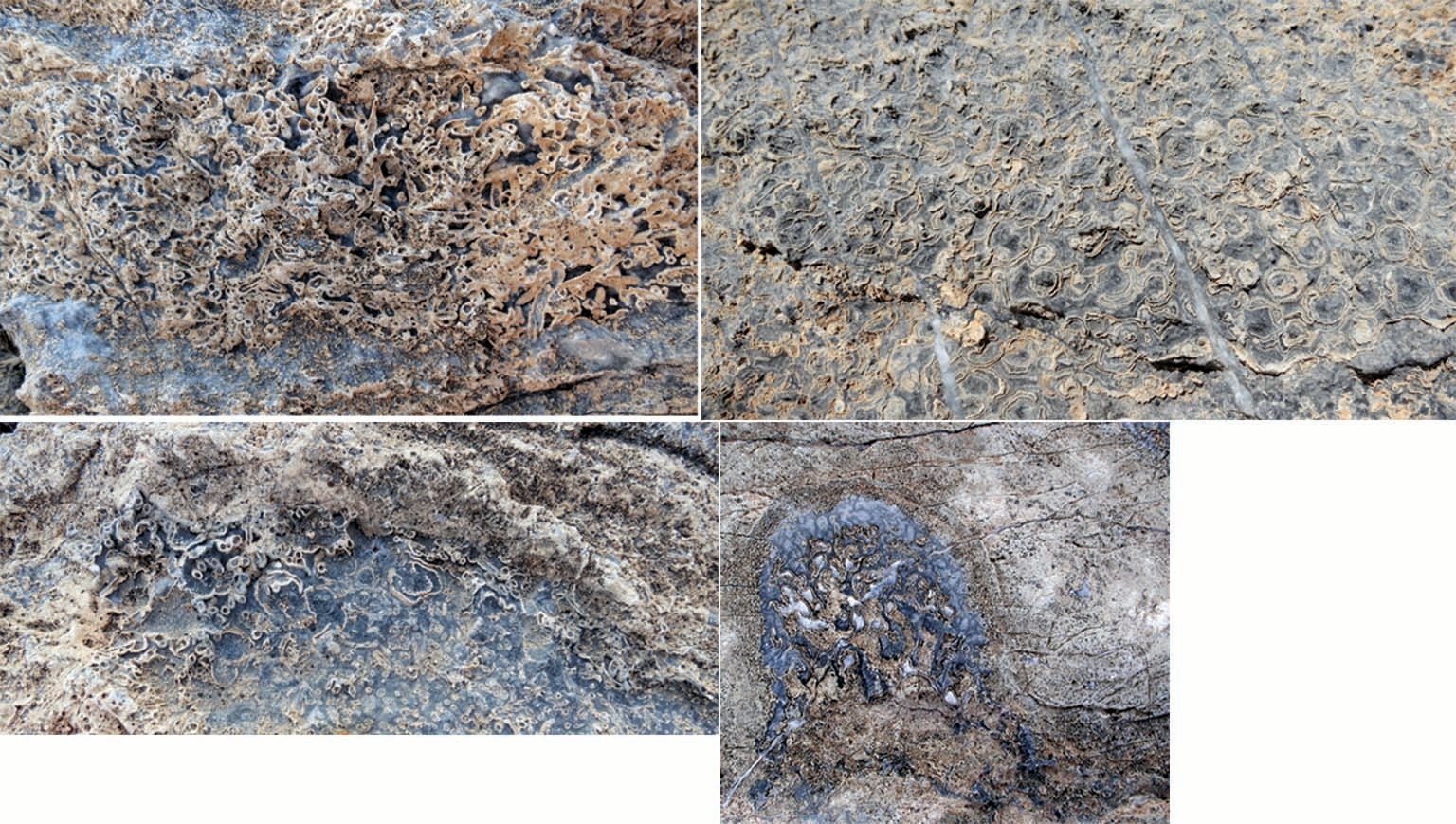
Fortunately for us, many new geochemical methods for estimating how much oxygen existed in these ancient seas have been developed in recent years. One especially powerful technique—Fe speciation—harnesses the characteristics of the various compounds of iron, which behave differently depending on whether oxygen is present or not. This method allows us to see at a local scale where—and when—there was enough oxygen to support complex life. Studies carried out using this approach have led to a broad consensus: dissolved oxygen in the oceans probably reached a threshold or series of thresholds during the Ediacaran that allowed animals to diversify by meeting their increasing metabolic demands as they became more mobile and active.
Scientists have now assembled sufficiently large geochemical data sets that we can reconstruct how oxygen was distributed not just at individual Ediacaran sites of a certain age but globally through time. This work reveals patterns throughout the Ediacaran and early Cambrian that differ considerably from today’s, with many areas showing a relatively thin veneer of well-oxygenated shallow waters laying atop a thicker wedge of deeper seawater that probably lacked oxygen altogether, a state known as anoxia.
These geochemical data also show that the boundary between the anoxic and oxic waters was very dynamic during this interval, rising and falling with shifting sea levels. Areas of shallow marine seafloor habitable to early animals were thus even more restricted than scientists expected—veritable oases of oxygenated water. If the evolutionary diversification that took place during the Ediacaran and Cambrian occurred under relatively low oxygen levels but with highly dynamic conditions that fluctuated on ecological, global and evolutionary timescales, how might these factors have shaped that extraordinary radiation?
Engine of innovation?
Periods of increased anoxia on the seafloor coincide with some well-known mass extinctions, such as the one that punctuated the Permian period 252 million years ago, killing off more than 90 percent of all marine species. But several major diversifications—including those in the Ediacaran-Cambrian, the Ordovician 100 million years later and the mid-late Triassic about 247 million years ago—began during long intervals of dynamic shallow marine anoxia. Considering these events, my colleague Doug Erwin of the Smithsonian Institution and I hypothesized that fluctuating oxygen conditions may have created critical opportunities for evolutionary innovation in soft-bodied animals.
It is far easier for animals to form a skeleton of limestone—the material that makes up the skeletons and shells of many modern marine creatures—when seawater oxygen levels exceed 10 micromoles per liter. Perhaps soft-bodied animals were only able to evolve these calcium carbonate skeletons once oxygen levels reached such a threshold, allowing formerly isolated oases to expand, connect and achieve stability on a global scale.
Much remains to be discovered about how life might have responded to changes in oxygen availability over evolutionary timescales. The response was probably complicated because animals were also contending with additional factors such as the rise of predation. And because feedbacks among individual organisms, ecosystems and the broader Earth system—which are largely unknown—would have also figured into the equation.
We have our work cut out for us. Dramatic changes in the regional processes that shaped Earth’s crust throughout the Ediacaran-Cambrian interval have produced many significant gaps in the geologic and fossil record. This means that we have to piece together our narrative about the rise of complex animals from data collected from a multitude of localities all over the world. The fact that many of the key Ediacaran localities are still poorly dated further complicates our task. We typically date rocks of this age by measuring the ratio of lead to uranium in zircon crystals found in nearby layers of ash from ancient volcanic eruptions. This is one of the few methods that can supply an absolute, radiometric age for a given rock. But frustratingly, many of our best-known successions lack these vital ash beds. As a result, we are unable to accurately correlate evolutionary changes that have occurred in different parts of the world, which is essential for creating a solid timeframe for our history of events. A prime example is China’s hotly debated Lantian Formation, which has yielded the oldest candidate animal fossils, but whose age could fall anywhere between 635 million and 590 million years.
Nevertheless, there are reasons for optimism. New ash beds are coming to light, and dating methods are being refined. For instance, the ash beds that many research groups use to calculate the ages of the Ediacaran fossils found in Namibia have recently been redated, and the youngest ones—those nearest the Precambrian-Cambrian boundary—have proved to be more than two million years younger than previously thought. This result raises important questions about how these fossils actually correlate with their counterparts in Newfoundland and Siberia, among other key localities. In addition, geochemists are developing new isotopic techniques and other methods that can bring our picture of oxygen conditions in this ancient world into sharper focus. And my team and others are finding new fossils in remote places that have gone largely unexplored until now, such as Siberia.
Sometime in the not so distant future, when we stand on those cliffs, surveying the vast forest below, we will have a far deeper understanding of this most extraordinary slice of time.